CATEGORY
Does Entry Into Dormant States During Cold Storage Impact Health Risk From E. coli O157:H7 on Lettuce?
The authors investigate whether cold storage time of leafy greens influences E. coli entry into dormant states and the corresponding risk of illness
By Joshua Ombaka Owade, Ph.D., Postdoctoral Research Associate, Department of Biosystem and Agricultural Engineering, Michigan State University (MSU); Jade Mitchell, Ph.D., Professor and Associate Department Chair, Department of Biosystems and Agricultural Engineering, MSU; and Teresa Bergholz, Ph.D., Associate Professor, Department of Food Science and Human Nutrition, MSU
SCROLL DOWN
Video credit: Rosendo Serrano Valera/Creatas Video+/Getty Images Plus via Getty Images
> SPOtliGHT
In many of the recent outbreaks of Escherichia coli (E. coli) O157:H7 linked to leafy greens, the majority of initial cases in the outbreak occurred in states on the East Coast or in the Midwest, as well as from the eastern provinces of Canada.1–4 During these outbreaks, it was frequently observed that illnesses were regionally distributed, even though the implicated product was distributed nationally. These observations suggested that product experiencing longer transport times prior to processing may be more likely to be associated with illness compared to leafy greens that are processed closely after harvest.
This hypothesis led the authors to investigate whether a key difference between source processing and forward processing—the length of time leafy greens are in cold storage prior to processing—influences E. coli O157:H7 entry into dormant states, and if so, how that may influence risk of illness.
Pathogen Response to Conditions in Pre- and Post-Harvest Environments
Bacteria respond to changing environmental conditions via alterations in gene and protein expression, metabolic activity, and/or cell wall structure. Adaptations in response to one condition, such as activation of proteins to combat reduced water availability on a leaf surface, can lead to improved survival at a subsequent stage of the produce processing chain, such as during washing with sanitizers.5 Adaptation responses have been commonly measured by monitoring changes in expression of stress response-associated genes.
Multiple studies have shown that adaptation of E. coli O157:H7 to lettuce surfaces involves activation of osmotic and oxidative stress responses, among others.5,6,7 Temperature shifts from ambient to refrigeration also activate adaptive responses, including the general stress response, osmotic stress response, and cold shock proteins.8–11 Although adaptations can improve survival, it is important to note that most stages of the lettuce supply chain lead to reductions in pathogen numbers.12
Another mechanism likely to contribute to pathogen survival through the lettuce supply chain is entering a dormant state that protects cells from harsh environments. These dormant states include the formation of persister cells and entry into the viable but nonculturable (VBNC) state, in response to adverse environmental conditions.13,14,15 E. coli O157:H7 persisters form on romaine lettuce plants under conditions that lead to cell die-off.16 Among Shiga toxin-producing E. coli, persister formation varies by strain and occurs to a greater extent under conditions that do not facilitate growth, such as in field water.17
E. coli O157:H7 strains from the recent leafy greens outbreaks entered into the persister and VBNC states when held for weeks in river water,18 one of the potential vehicles for transmission of the pathogen to crops. It is clear that E. coli O157:H7 can enter into dormant states in environments relevant to the pre-harvest lettuce growing environment. Dormant states can also be induced on post-harvest lettuce, where the population of E. coli O157:H7 on lettuce leaves stored at 8 °C (46.4 °F) shifted to the VBNC state, losing culturability after 5 days, while still producing Shiga toxin.19 This suggests that cells in the VBNC state, while not detectable with standard methods, may retain their virulence potential.
E. coli O157:H7 Survival Dynamics Impact Risk of Consumption
The exposure of humans to E. coli O157:H7 at the point of consumption is determined by the microbial dynamics on lettuce as the product moves through the supply chain (Figure 1). Die-off of E. coli O157:H7 on preharvest lettuce after deposition is typically bi-phasic, and levels of reduction are influenced by environmental and weather conditions including temperature, relative humidity (RH), and light.20,21
FIGURE 1. The exposure of humans to E. coli O157:H7 at the point of consumption is determined by the microbial dynamics on lettuce as the product moves through the supply chain (Image credit: fcafotodigital/E+ via Getty Images)
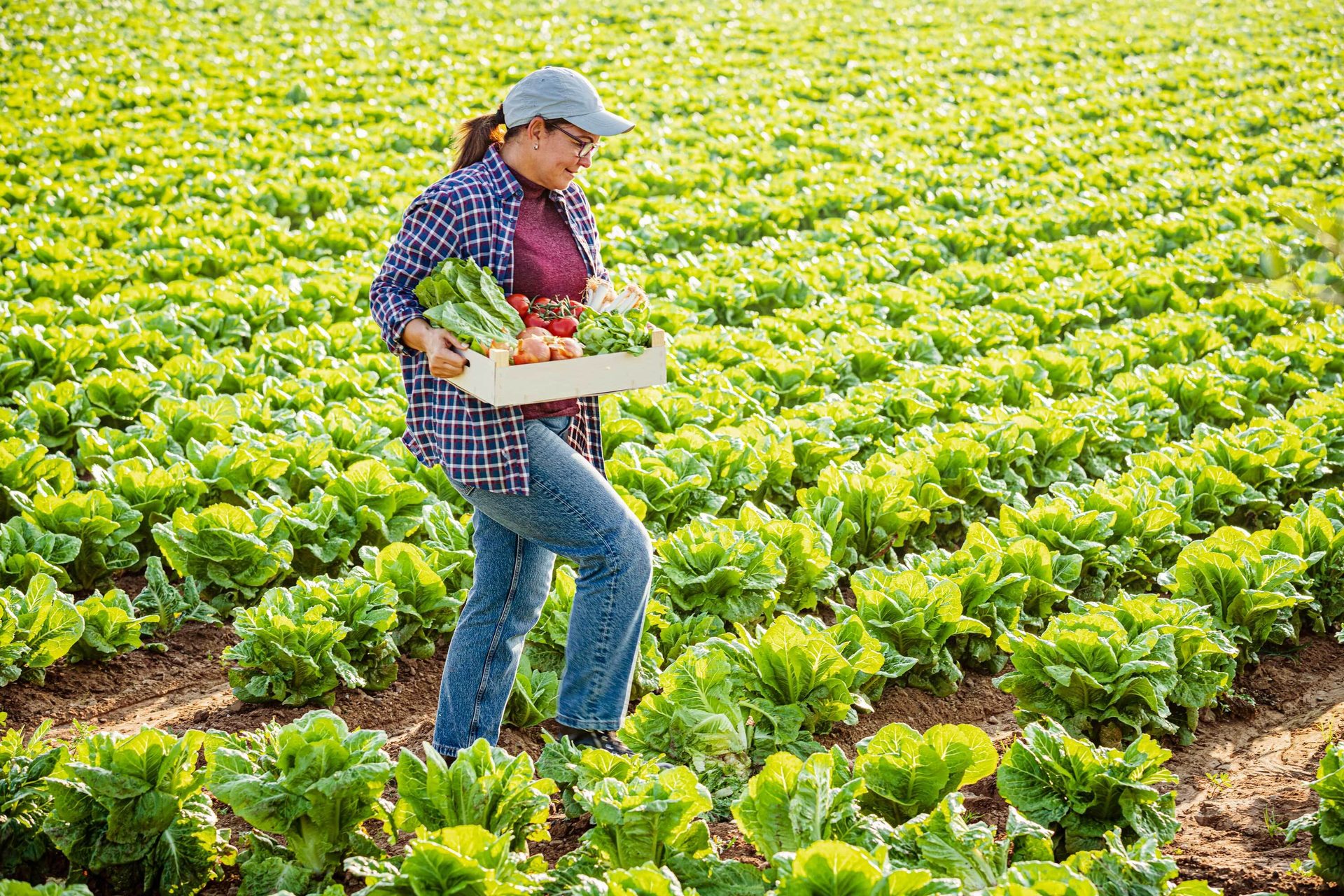
Several studies have elucidated the individual and isolated effects of environmental conditions. However, the interactions of environmental conditions on E. coli die-off were found to explain more of the variance compared to the individual effects.21 Exposure to pre-harvest conditions influences the post-harvest E. coli dynamics, including at the processing stage. For example, relative humidity levels and length of time associated with pre-harvest of lettuce plants influenced subsequent post-harvest tolerance of E. coli O157:H7 to chlorine.5
Much work has been done to characterize die-off of microbial cells in-field;20,21 however, the assuming uniform distribution of die-off (such as due to chlorine treatment22) may underestimate the survival of the pathogens, especially in instances where there is high chlorine tolerance. In many cases, predictions of die-off do not include these interaction effects or all stages of the lettuce supply chain. Holistic inclusion of these factors, encompassing the entire supply chain, will be essential to improve predictions regarding pathogen die-off on lettuce from farm to fork.
“In addition to estimating the risk of adverse health outcomes, quantitative microbial risk assessment is used in assessing efficacy of interventions such as temperature control and identifying important factors that influence risk.”
QMRA and Risk of Human Illness: A Tool for Quantifying Microbial Risks in Food
Quantitative microbial risk assessment (QMRA) is a tool used in establishing human health risks arising from microbial contamination of food, including lettuce. In addition to estimating the risk of adverse health outcomes, QMRA is used in assessing the efficacy of interventions such as temperature control and identifying important factors that influence risk. The QMRA framework comprises four steps:
- Hazard identification
- Exposure assessment
- Dose response assessment
- Risk characterization.
The first step is hazard identification—i.e., determining the types of pathogens, produce, and scenarios that can potentially present health risks to the consumers.
The second step, exposure assessment, explores how much of the pathogen the consumer ingests when eating lettuce. The models in this step range from simple to complex, as some foods can have multiple steps, multiple stakeholders, and several conditions that affect the survival of the pathogen. For instance, during harvesting of field-bagged romaine lettuce hearts, the head of lettuce is cut approximately 2 inches above the ground, limiting the contact between the produce and the soil and thereby reducing the chance of pathogen transfer onto the produce. On the other hand, romaine lettuce leaves are harvested by cutting the produce at the base. Contact between the soil, harvesting equipment, and the produce can result in the transfer of E. coli O157:H7 to the leaves (Figure 2).
FIGURE 2. Contact between the soil, harvesting equipment, and the produce can result in the transfer of E. coli O157:H7 to the leaves (Image credit: Manjurul/iStock/Getty Images Plus via Getty Images)
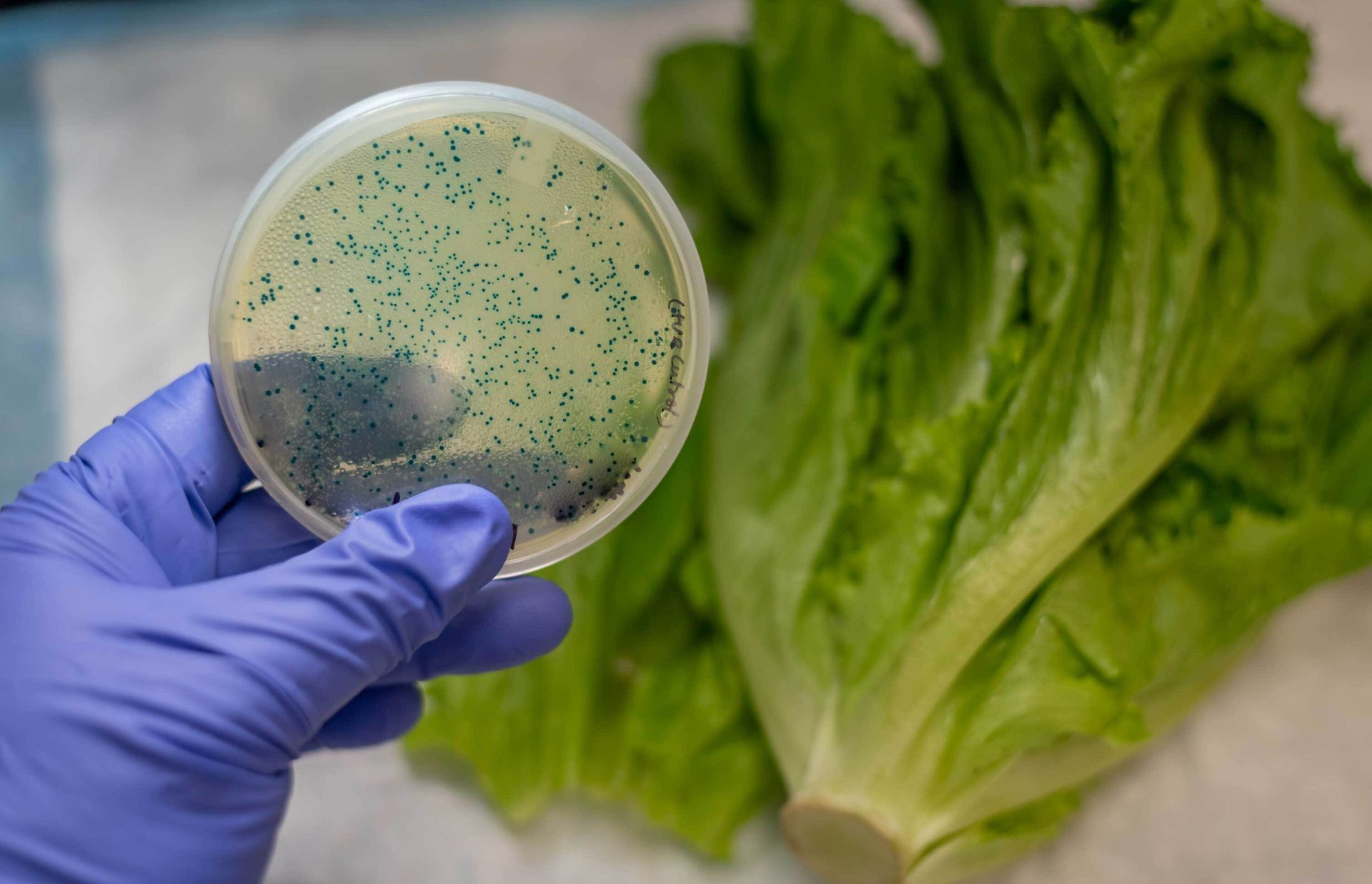
The third step, dose-response assessment, addresses the question of how the risk of a particular adverse health outcome in a person relates and changes based on how much of a specific pathogen is consumed.
Finally, risk characterization quantifies the magnitude of risks of the adverse health outcome from consumption of lettuce contaminated with a specific pathogen in a population or sub-population. This step combines the inputs from the exposure and dose-response assessment. Probabilistic QMRA distributions are used to explain the uncertainty in the quantities used in each step and, ultimately, to generate a distribution of the risk outcome so that the likelihood of outcomes can also be communicated.
What is So Important in the New Risk Model?
Several published models describe the microbial risks associated with E. coli O157:H7 contamination in lettuce. However, the authors have developed a new model for three key reasons:
- Existing models typically use first-order kinetics, which assume a constant die-off rate, to describe the inactivation of E. coli O157:H7 along the exposure pathway of leafy greens.23,24,25 However, our previous study21 demonstrated that E. coli O157:H7 inactivation in leafy greens follows nonlinear patterns rather than a constant die-off rate. The inactivation curves exhibit various forms, including linear trends, shouldering, tailing, and sigmoidal shapes with both shouldering and tailing phases, with the latter three describing the inactivation using varying die-off rates (Figure 3).
- Standard culturing or molecular detection methods do not account for the presence of VBNC E. coli O157:H7 cells. Industry-standard plate count techniques can only detect culturable cells, potentially underestimating the survival of the pathogen. VBNC cells, although undetectable by these methods, can regain culturability under favorable conditions and pose a risk to consumers.
- Many existing models assume no forward processing delays. However, our mapping of the lettuce value chain revealed that, after harvesting, produce is transported to cooling facilities, often with a time lag. Proper refrigeration is recommended during this period; however, temperature abuse can occur, allowing pathogen growth and increasing risks at consumption.
The new model addresses these critical gaps, providing a more accurate assessment of E. coli O157:H7 risks in lettuce.
FIGURE 3. Trends of Inactivation Curves Fitted to the Die-Off Data of E. coli O157:H7 in Leafy Greens
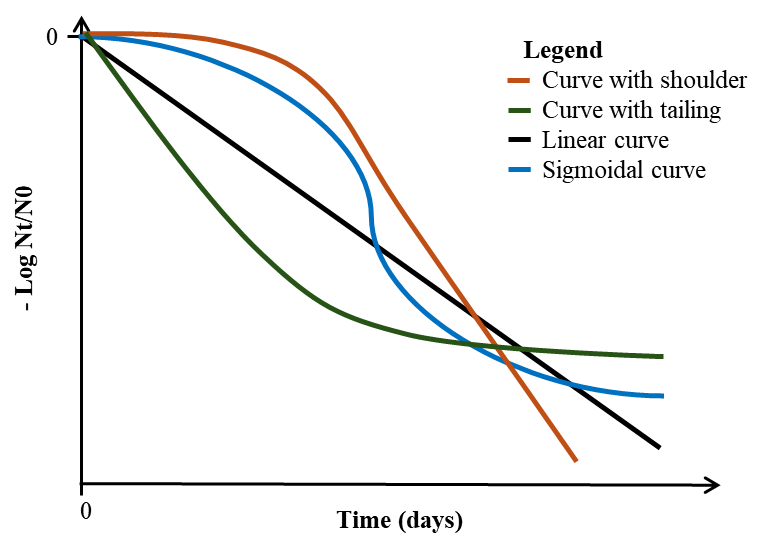
The fitted microbial data is in log reduction computed from Nt as microbial population at time t and N0 as the initial concentration.
Linking Bacterial Behavior Along the Exposure Pathway to Model Inputs for QMRA
The exposure assessment tracks lettuce through the supply chain, evaluating how each step influences microbial behavior and the eventual dose in the consumed portion. As the produce moves from production to consumption (Figure 4), the process begins with identifying contamination routes through which E. coli O157:H7 reaches the produce.
FIGURE 4. QMRA Framework Accounting for the Impact of Forward Processing Delays on the Risk of STEC O157:H7 in Romaine Lettuce
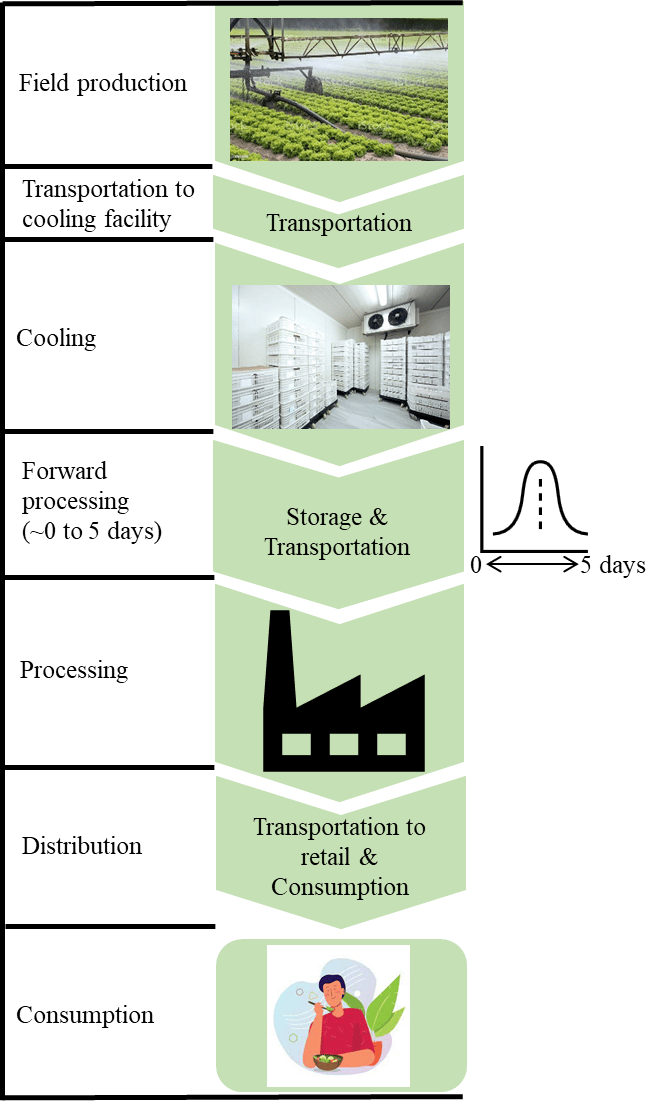
In-Field Contamination and Die-Off
Contamination in the field can occur during irrigation and harvesting. The transfer of E. coli O157:H7 cells from irrigation water to lettuce depends on water retention and pathogen attachment. Industry guidelines, such as the California Leafy Greens Marketing Agreement (LGMA), recommend that any single irrigation water sample should not exceed 235 most probable number (MPN)/100 mL of generic E. coli to minimize contamination risks.26 Following irrigation, during the holding period before harvest, environmental stressors—including light, temperature, and humidity—contribute to microbial die-off.12
Soil serves as a reservoir for E. coli O157:H7, and direct contact between soil, harvesting equipment, and lettuce can facilitate pathogen transfer. Additionally, exposure to environmental stressors during field production may induce the formation of VBNC cells, resulting in a loss of culturability.19
Post-Harvest Handling and Processing
After harvesting, lettuce is transported under refrigerated conditions to limit deterioration and microbial growth. While low temperatures promote microbial die-off, they can also induce the VBNC state in E. coli O157:H7. Romaine hearts are transported directly to retail, whereas shredded and packaged lettuce undergo further processing including washing, shredding, and packaging.
Washing with sanitizers, such as chlorine, reduces pathogen load by removing contaminants and inactivating E. coli O157:H7, aiming to prevent further contamination; however, subsequent processing steps such as shredding, centrifugation for water removal, and packaging, introduce opportunities for cross-contamination.27 E. coli O157:H7 cells may transfer from contaminated leaves to equipment surfaces, increasing the risk of spreading to uncontaminated batches.
Retail, Distribution, and Consumer Handling
At the retail and consumer handling stages, temperature plays a critical role in determining microbial survival.12 Favorable conditions can promote pathogen proliferation, whereas refrigeration enhances die-off. However, variability in retail and home storage temperatures, as well as exposure duration, significantly influences E. coli O157:H7 growth or reduction.
“The goal of our research was to understand how harvest temperature and length of cold storage prior to processing impacts E. coli O157:H7 physiology, and if those changes impact the risk of illness due to consumption of romaine lettuce.”
E. coli O157:H7 on Lettuce During Cold Storage
Assessing the Impact of Harvest Temperature and Cold Storage on Microbial Dynamics
The authors conducted an experiment to simulate the exposure of E. coli O157:H7 cells on lettuce to cold stress over a five-day period. Lettuce, grown under greenhouse conditions for 4–6 weeks, was placed in a plant growth chamber for 4 hours at temperatures of 9 °C (48.2 °F) and 17 °C (62.6 °F) to reflect a range of industry-standard harvesting conditions. Following this pre-harvest temperature exposure, the produce was inoculated with actively growing E. coli O157:H7 cells and then transferred to a cold storage chamber for 5 days. Daily sampling was conducted to quantify culturable cells, using culture-based methods, as well as to quantify those in the persister and VBNC states, using quantitative polymerase chain reaction (PCR) with propidium monoazide.
A Probabilistic QMRA for E. coli O157:H7 on Lettuce
We conducted a probabilistic QMRA to establish the risk of illness due to E. coli O157:H7 in an individual from consuming either field-bagged romaine hearts or shredded lettuce (subjected to shredding, washing in water containing chlorine, and packaged). Our exposure model mapped the supply chain of lettuce through production, processing, transport and distribution, and storage (Figure 2). The risk of illness per serving per person was computed by integrating information from the exposure assessment and the dose-response model. To estimate the annual risk, the number of eating occasions was incorporated. Additionally, we evaluated the effect of physiological changes on the final risk by modeling different scenarios that accounted for VBNC transition rates across various cold storage durations.
Cold Stress-Induced Dormancy and Tolerance in E. coli O157:H7 Cells
As other previous studies observed,16,19 our study also showed that storage of lettuce under cold stress led to declining culturable fraction of the E. coli O157:H7 cells. The cells transitioned into dormancy during cold storage; however, longer exposure did not translate into increasing rates of formation of both VBNC and persister cells. We also established that lettuce harvested at colder temperatures had more E. coli O157:H7 cells transition into dormant states than in produce harvested at warmer conditions. Since conventional plate count techniques can possibly miss out in accounting for the VBNC cells, we computed the switch rates as the ratio of VBNC to culturable counts in CFU over the cold storage periods and used them to simulate the effect of cold stress on risks in our QMRA.
Accounting for Physiological Transitions of E. coli O157:H7 Did Not Increase the Predicted Risk
The goal of our research was to understand how harvest temperature and length of cold storage prior to processing impacts E. coli O157:H7 physiology, and if those changes impact the risk of illness due to consumption of romaine lettuce. We know that E. coli O157:H7 can enter into dormant states, which can be classified as the persister state, as well as viable but non-culturable (VBNC). We wanted to know if lettuce harvest temperature and length of cold storage influenced the number of cells in the dormant state, and if that made them more tolerant to subsequent stressors such as chlorine, which would be encountered during processing. We combined our laboratory data with data from published literature and conducted a quantitative microbial risk assessment (QMRA) to determine if the risk of illness changed based on the length of time in refrigerated storage prior to processing.
We established that there was no difference in the risk of illness when we compared the adverse health outcomes from consuming the field-bagged romaine hearts to those of shredded and packaged lettuce. Storage under cold stress induced the formation of VBNC cells on the produce during storage. We did not establish any increase in the VBNC switch rates over the cold period, and we recorded no difference resulting from harvesting in either warm or cooler harvesting temperatures. The risk of illness did not increase with increasing period under cold storage of either field-bagged romaine hearts or shredded lettuce.
References
- Waltenburg, M.A., C. Schwensohn, A. Madad, S.L. Seelman, V. Peralta, S.E. Koske, et al. "Two multistate outbreaks of a reoccurring Shiga toxin-producing Escherichia coli strain associated with romaine lettuce: USA, 2018–2019." Epidemiology and Infection 150, no. 16 (2021).
- Irvin, K., S. Viazis, A. Fields, S. Seelman, K. Blickenstaff, E. Gee, et al. "An overview of traceback investigations and three case studies of recent outbreaks of Escherichia coli O157:H7 infections linked to romaine lettuce." Journal of Food Protection 84, no. 8 (2021): 1340–1356.
- Marshall, K.E., A. Hexemer, S.L. Seelman, M.K. Fatica, T. Blessington, M. Hajmeer, et al. "Lessons Learned from a Decade of Investigations of Shiga Toxin-Producing Escherichia coli Outbreaks Linked to Leafy Greens, United States and Canada." Emerging Infectious Diseases 26, no. 10 (2020): 2319–2128.
- Bottichio, L., A. Keaton, D. Thomas, T. Fulton, A. Tiffany, A. Frick, et al. "Shiga Toxin-Producing Escherichia coli Infections Associated with Romaine Lettuce—United States, 2018." Clinical Infectious Diseases 71, no. 8 (2020):E323–30.
- Tyagi, D., A.L. Kraft, S.L. Smith, S.E. Roof, J.S. Sherwood, M. Wiedmann, et al. "Pre-harvest survival and post-harvest chlorine tolerance of enterohemorrhagic Escherichia coli on lettuce." Toxins 11, no. 11 (2019): 1–15.
- Kyle, J.L., C.T. Parker, D. Goudeau, and M.T. Brandl. "Transcriptome analysis of Escherichia coli O157:H7 exposed to lysates of lettuce leaves." Applied and Environmental Microbiology 76, no. 5 (2010): 1375–1387.
- Van der Linden, I., B. Cottyn, M. Uyttendaele, G. Vlaemynck, M. Heyndrickx, M. Maes, et al. "Microarray-Based Screening of Differentially Expressed Genes of E. coli O157:H7 Sakai During Preharvest Survival on Butterhead Lettuce." Agriculture 6, no. 1. (2016): 1–22.
- Carey, C.M., M. Kostrzynska, and S. Thompson. "Escherichia coli O157:H7 stress and virulence gene expression on Romaine lettuce using comparative real-time PCR." Journal of Microbiological Methods 77, no. 2 (2009): 235–242.
- Allen, K.J., D. Lepp, R.C. McKellar, and M.W. Griffiths. "Examination of stress and virulence gene expression in Escherichia coli O157:H7 using targeted microarray analysis." Foodborne Pathogens and Disease 5, no. 4 (2008): 437–447.
- Kennedy, N.M., N. Mukherjee, and P. Banerjee. "Escherichia coli O157:H7 cells exposed to lettuce leaf lysate in refrigerated conditions exhibit differential expression of selected virulence and adhesion-related genes with altered mammalian cell adherence." Journal of Food Protection 79, no. 7 (2016): 1259–1265.
- Sharma, M., S. Lakshman, S. Ferguson, D.T. Ingram, Y. Luo, and J. Patel. "Effect of modified atmosphere packaging on the persistence and expression of virulence factors of Escherichia coli O157:H7 on shredded iceberg lettuce." Journal of Food Protection 74, no. 5 (2011): 718–726.
- Owade, J.O., T.M. Bergholz, and J. Mitchell. "A review of conditions influencing fate of Shiga toxin-producing Escherichia coli O157:H7 in leafy greens." Comprehensive Reviews in Food Science and Food Safety 23 (2024): 1–21.
- Kussell, E., R. Kishony, N.Q. Balaban, and S. Leibler. "Bacterial persistence: A model of survival in changing environments." Genetics 169, no. 4 (2005): 1807–1814.
- Ayrapetyan, M., T. Williams, and J. Oliver. "Relationship Between the Viable but Nonculturable State and Antibiotic Persister Cells." Journal of Bacteriology 200, no. 20 (2018): 1–15.
- Kim, J.S., N. Chowdhury, R. Yamasaki, and T.K. Wood. "Viable but non-culturable and persistence describe the same bacterial stress state." Environmental Microbiology 20, no. 6 (2018): 2038–2048.
- Munther, D.S., M.Q. Carter, C.V. Aldric, R. Ivanek, and M.T. Brandl. "Formation of Escherichia coli O157: H7 persister cells in the lettuce phyllosphere and application of differential equation models to predict their prevalence on lettuce plants in the field." Applied and Environmental Microbiology 86, no. 2 (2020).
- Thao, S., M.T. Brandl, and M.Q. Carter. "Enhanced formation of Shiga toxin-producing Escherichia coli persister variants in environments relevant to leafy greens production." Food Microbiology 84 (2019).
- Carter, M.Q., D. Carychao, and J.L. Bono JL. "Comparative analyses of persistence traits in Escherichia coli O157:H7 strains belonging to different clades including REPEXH01 and REPEXH02 strains." Frontiers in Microbiology 15 (2024).
- Dinu, L.D. and S. Bach. "Induction of viable but nonculturable Escherichia coli O157:H7 in the phyllosphere of lettuce: A food safety risk factors." Applied and Environmental Microbiology 77, no. 23 (2011): 8295–8302.
- Belias, A.M., A. Sbodio, P. Truchado, D. Weller, J. Pinzon, M. Skots, et al. "Effect of weather on the die-off of Escherichia coli and attenuated Salmonella enterica serovar typhimurium on preharvest leafy greens following irrigation with contaminated water." Applied and Environmental Microbiology 86, no. 17 (2020): 1–25.
- Owade, J.O., T.M. Bergholz, and J. Mitchell. "A meta-analysis of factors influencing the inactivation of Shiga toxin-producing Escherichia coli O157:H7 in leafy greens." Comprehensive Reviews in Food Science and Food Safety 23 (2024): 1–22.
- Mokhtari, A., H. Pang, S. Santillana Farakos, G.R. Davidson, E.N. Williams, and J.M. Van Doren. "Evaluation of potential impacts of free chlorine during washing of fresh-cut leafy greens on Escherichia coli O157:H7 cross-contamination and risk of illness." Risk Analysis 42, no. 5 (2022): 966–988.
- Mokhtari, A., D. Oryang, Y. Chen, R. Pouillot, and J. Van Doren. "A Mathematical Model for Pathogen Cross-Contamination Dynamics during the Postharvest Processing of Leafy Greens." Risk Analysis 38, no. 8 (2018): 1718–1737.
- Pang, H., E. Lambertini, R.L. Buchanan, D.W. Schaffner, and A.K. Pradhan. "Quantitative microbial risk assessment for Escherichia coli O157:H7 in fresh-cut lettuce." Journal of Food Protection 80, no. 2 (2017): 302–311.
- Hamilton, A.J., F. Stagnitti, R. Premier, A.M. Boland, and G. Hale. "Quantitative microbial risk assessment models for consumption of raw vegetables irrigated with reclaimed water." Applied and Environmental Microbiology 72, no. 5 (2006): 3284–3290.
- Western Growers. "Commodity specific food safety guidelines for the production of and harvest of lettuce and leafy greens." California Leafy Greens Marketing Agreement (LGMA). 2021.
- Pérez Rodríguez, F., D. Campos, E.T. Ryser, A.L. Buchholz, G.D. Posada-Izquierdo, B.P Marks, et al. "A mathematical risk model for Escherichia coli O157:H7 cross-contamination of lettuce during processing." Food Microbiology 28, no. 4 (2011): 694–701.
Joshua Owade, Ph.D. is a Postdoctoral Research Associate and the Coordinator of the Quantitative Microbial Risk Assessment Interdisciplinary Vehicle (QMRA IV) Program at Michigan State University (MSU). His research focuses on risk modeling and predictive microbiology in food matrices, with an emphasis on enhancing food safety through quantitative modeling of microbial behavior along the produce supply chain.
Jade Mitchell, Ph.D. is Professor and Associate Chair in the Department of Biosystems and Agricultural Engineering at MSU. Her research expertise is in human health risk analysis, specifically understanding how chemical and microbial stressors from diverse environmental exposures lead to adverse human health outcomes. She uses quantitative analysis and statistical and mechanistic modeling to characterize risks to support risk management decision-making, including engineering design and environmental policy.
Teresa Bergholz, Ph.D. is an Associate Professor in the Department of Food Science and Human Nutrition at MSU. Her research expertise is in food safety microbiology, focused on understanding foodborne pathogen responses to environmental stressors in the food supply. She utilizes genomics, transcriptomics, and phenotypic assays to evaluate pathogen behavior and develop interventions for reducing pathogens in the food supply.