SANITATION
By Larry Keener, CFS, PA, President and CEO, International Product Safety Consultants
Hydroxyl Radicals for a Radical Advantage in Food Processing Hygiene: A Survey of Biocides Used by the Food Industry—Part 1
The use of hydroxyl radical air cleaners is a unique and valuable addition to the food industry's methods of minimizing pathogens in air and on surfaces
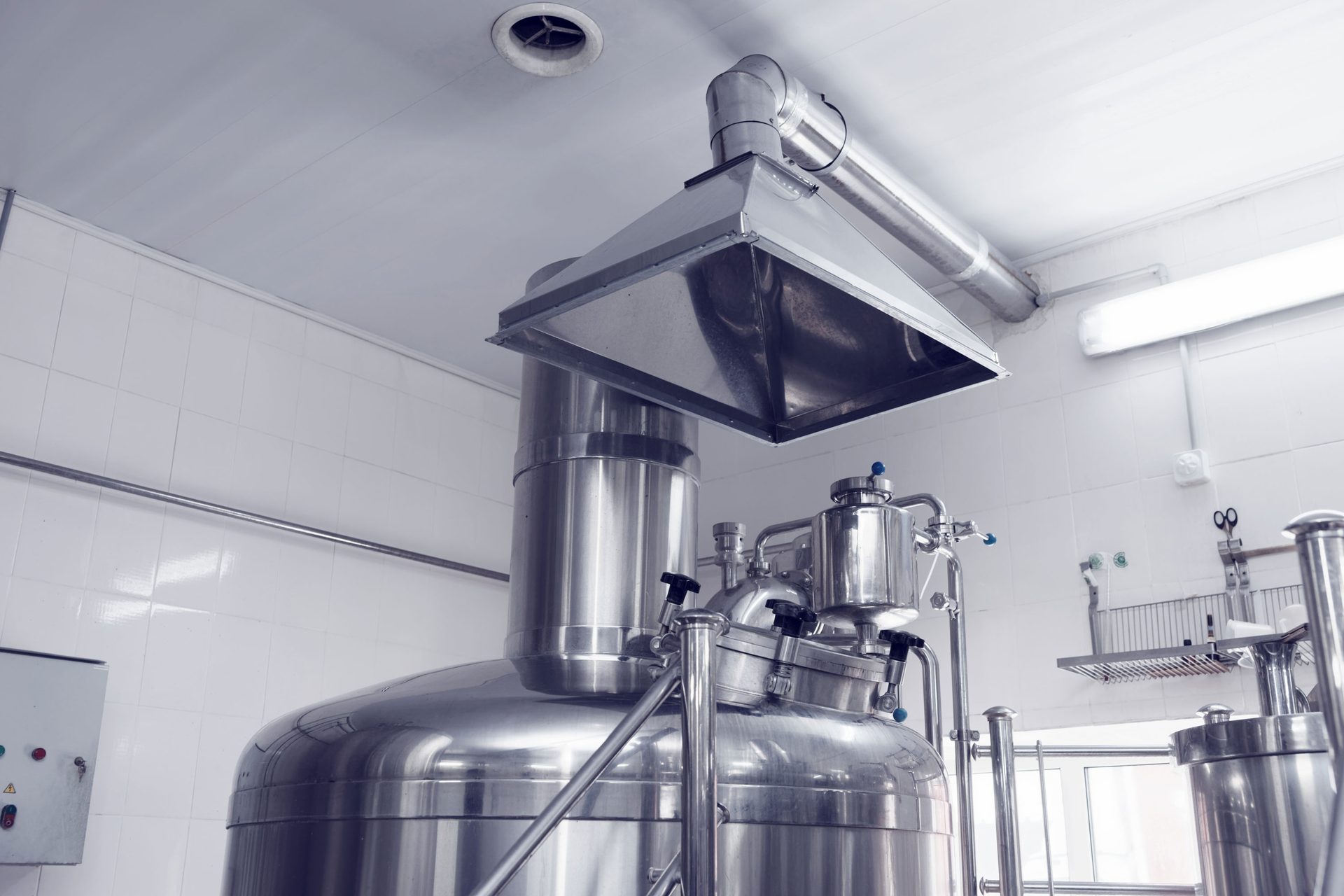
Image credit: Kondor83/iStock/Getty Images Plus via Getty Images
SCROLL DOWN
A number of biocides—including chlorine dioxide, hydrogen peroxide, ozone, etc.—have a long history of use in food plant hygiene control programs. Many of these compounds are toxic to humans and cannot be used during normal production-related activities. Application of these chemicals requires specialized training, and personnel are frequently required to wear personal protective equipment (PPE) when handling them. These costly extra precautions and the loss of asset utilization are not incurred when using hydroxyl radicals for ambient air and surface disinfection.
Mechanical devices generate reactive molecules to clean air and surfaces, including airborne oxidizing chemicals, ions, and hydroxyl radicals. Recent lab-based and real-world case studies have shown that the use of hydroxyl radicals, at the appropriate concentration, offers a proven, highly effective, safe, and scalable approach to food plant hygiene and food safety. This outcome can be achieved by devices that use ultraviolet (UV) energy to generate hydroxyls from water vapor, turning the ambient air into a mechanism for cleaning. The sun's UV energy generates similar concentrations of hydroxyls in the atmosphere to sanitize and cleanse the environment.
Hydroxyl Radical Background and Use in Food Plant Sanitation
Two types of hydroxyl radicals (OH) are found in nature: biological and atmospheric. Biological hydroxyls are formed by immune system-generated blood cells at infection sites when the normal infection-fighting action of lymphocytes is inadequate. The hydroxyl radical cleaves the covalent bonds in the proteins, carbohydrates, and lipids that make up the cell walls of pathogens, thereby destroying the cell membranes. This reaction is short-lived (approximately 10-9 sec.) and designed by the body to react very rapidly, with no selectivity. It occurs with almost every type of molecule found in living cells including sugars, amino acids, phospholipids, DNA, organic acids, and fatty acids.1,2
Atmospheric hydroxyls are present throughout the troposphere, where they are formed by the photolysis of O3 by the sun's UV energy greater than 315 nanometers (nm); shorter UV wavelengths are filtered out by the stratospheric ozone layers.3,4 This forms singlet oxygen O(1D), which reacts with water (H2O) to form two OH radicals. OH can also be formed directly by the UV scission of H2O at wavelengths below 200 nm and by the UV dissociation of hydrogen peroxide (H2O2).1,3,4 Typical steady-state OH concentrations on a sunny day are 3–10 million molecules per cubic centimeter (cm3).
Even at these low concentrations, which are less than one part per billion, OH is the primary oxidant for nearly all source gases emitted—both natural and manmade. The hydroxyl radical is the second-most reactive oxygen species known after elemental fluorine, and it reacts within milliseconds by removing hydrogen atoms from nearly all chemicals and the cell walls of microorganisms. The hydroxyl radical is often referred to as the "detergent" of the troposphere because it reacts with many pollutants, biological materials, and microorganisms, often acting as the first step to their removal.3,4,5 Due to its reactive properties, hydroxyl radicals are an effective method for decontaminating ambient air and myriad other hard surfaces and porous materials, including fabric and paper, within a food production environment.
Atmospheric hydroxyls do not occur naturally indoors. Technologies have been developed to generate OH for sanitizing indoor air and food contact surfaces. The use of OH reactive air cleaners is a unique and valuable addition to the food industry's standard methods of minimizing pathogens in air and on surfaces. Source control and ventilation are the primary control methods in use by the industry. These techniques often use chemical and UV sanitizing systems as complementary and synergistic.
Reactive air cleaners represent a new generation of systems to optimize the quality of indoor air and feature the generation of reactive agents to clean air and surfaces that include aerosolized and volatile oxidizing chemicals, ions, and hydroxyl radicals. The most promising of these reactive agents are airborne OH because they are safely generated by the action of UV light on water vapor, are highly reactive, can inactivate pathogens as well as volatile organic compounds (VOCs), can reach all surfaces, and are safe for use in occupied spaces. Other types of reactive air cleaning methods and chemicals are also discussed in this article.
Tackling Decontamination with OH and Ambient Air
Ambient air is a recognized source of microbial contamination in food production facilities. The aerial microbial load and its diversity will be impacted by the type of microbial contamination on the raw intake materials, the airflow characteristics within the facility, relative humidity, and the movement of people and machines, among other elements. The microbiota present in the air may also have the potential to cause foodborne disease or reduce the shelf life of the food products. Hence, for companies manufacturing ready-to-eat (RTE) food items, it is essential that ambient air is given appropriate consideration in the company's risk assessments for food safety assurance.
Both by volume and by surface area, ambient air is the largest food contact surface (Zone 1) in the food processing industry. Ambient air is high risk and a serious potential source of biological contamination, including contamination by pathogenic bacteria. Frequently, it is also the most neglected and under-studied element of a food safety plan, and is often overlooked in the risk calculations for many food processing operations.

“Although necessary, the activities of the environmental monitoring program have a negative impact on productivity—i.e., the facility's production assets are idled while these essential sanitation activities are implemented.”
The food processing industry spends many thousands of dollars annually to study the environmental conditions within food processing establishments. Often, government regulations are the impetus for this spending behavior. To protect public health, it is the government's expectation that human food is prepared, packed, and held under sanitary conditions. It is reported that even the smallest companies in the industry spend upward of $10,000 USD annually on environmental monitoring programs (EMPs). These funds are typically spent on testing (swabbing) and inspecting floors, walls, drains, and food processing equipment. The EMP seeks evidence of microbial contamination, or verification that an item of equipment is clean and hygienic. A positive finding for bacteria, yeasts, or mold in food facilities may be due to the microbial quality of inbound raw materials, open drains, high levels of moisture, facility layout and design, or poor hygienic practices. Although necessary, the activities of the EMP have a negative impact on productivity—i.e., the facility's production assets are idled while these essential sanitation activities are implemented. Activities that anticipate and then mitigate sources of potential contamination can alleviate this loss of asset utilization and result in productivity gains. The use of hydroxyl radicals is a proven method for reducing the biological burden of ambient air,1 and thereby a net overall reduction of biological contamination within the entire production operation.
Case Histories of Food Contamination Caused by Unhygienic Air
The food safety canon provides an abundance of evidence confirming that ambient air is a viable mechanism in disseminating dangerous microbial contaminants. As far back as 1986, the U.S. Food and Drug Administration (FDA) published a status report titled, "Dairy Products Initiatives Preliminary Status Report."4,6 In that document, it was noted, "Airborne contamination is strongly suspected as the cause of some pathogenic contamination. A comprehensive assessment of the air supply and utilization, both processing and ventilation air, should be conducted."
Many other researchers over the years have reported recovering viable Listeria monocytogenes from the air within food production facilities.7 Certainly, for companies producing RTE foods, it is in their best interest and the interest of public health to have a fundamental understanding of the ambient air quality contained within the boundaries of their production operations.
As a process authority, the author has always stipulated hygienic air in the filling and packaging operations of companies that produce cold-mixed and cold-filled items, such as acidic cooking aids and condiments. I consider ambient air quality an imperative for avoiding premature spoilage in these products. I have also investigated a spoilage outbreak with a leading jam and jelly manufacturer, and concluded that the cause of spoilage was contaminated air. In this incident, mold found within the company's air handling and air distribution systems was identified as the spoilage organism.
In a June 2024 report from FDA, it was shown that Shiga toxin-producing Escherichia coli (STEC) contamination in leafy greens was caused by an airborne mechanism. In the FDA study, from 2019 through 2024, it was found that "air samples collected during that interval yielded positive viable pathogen results, indicating that bacteria can survive in the air and that dust can act as a transfer mechanism for both pathogens and indicator organisms from adjacent and nearby land to water, soil, and plant tissue."8
Food processing factories are susceptible to the spread of airborne viruses and other microbes. The temperature and humidity requirements, along with the production processes, are ideal for supporting the survival of airborne microorganisms. This means that airborne bacteria, viruses, yeasts, and molds can survive longer in food plant air than they would in many other facilities. Some pathogenic bacteria such as Salmonella, E. coli, and Listeria are able to survive in the air and have the potential to contaminate food products due to their high tolerance to environmental factors.9
Air Filtering and Change Rate Recommendations
These findings from FDA and others have major implications for companies engaged in the production and distribution of RTE food items. Historically, when food companies have focused on ambient air quality as a component of food safety assurance, they have relied almost exclusively on the minimum efficiency rating value (MERV) of their HVAC system, coupled with the air change rate per hour (ACH), as the primary protection against airborne modes of product contamination.
According to the American Society of Heating, Refrigerating, and Air-Conditioning Engineers (ASHRAE), the MERV rating of air filters used in food manufacturing facilities depends on the type of food being processed and the regulations that apply.10 The Centers for Disease Control and Prevention (CDC) and ASHRAE recommend a minimum rating of MERV 13, but prefer MERV 14. Filters with a MERV rating of 14 or higher can help capture bioaerosols. Highly micro-sensitive products, such as RTE foods, may need final filters with a MERV rating of 14 to 15, which can filter particles as small as 1.0 micron or less with greater than 99 percent efficiency.11 In my own practice, for the past 30 years, I have routinely recommended MERV 15 or higher for my clients engaged in the production of RTE food products.
FDA and the U.S. Department of Agriculture's Food Safety and Inspection Service (USDA-FSIS) state that food should be produced in a manner that does not compromise public health, which may include addressing hazards associated with air quality. At present, USDA-FSIS and FDA do not enforce ambient air sampling monitoring frequencies or define acceptable levels of contamination for either allergens or biological agents.
Utilizing higher-rating MERV filters does come with the downside of potentially requiring larger air handling units due to the reduction of airflow through the filter media, as well as the additional wear and tear and energy costs associated with the HVAC equipment. Lastly, the filter media itself becomes a collection point for pathogens and requires frequent replacement.
The air change rate per hour is another parameter that is frequently considered when discussing ambient air quality in the food plant. ACH is the measure of the frequency during which the total volume of air within a defined space is completely exchanged with makeup air or fresh air. ACH, according to ASHRAE standard 62.1, is the number of times per hour that all of the air in a space is pulled through the HVAC system.11 ACH in food production facilities will vary depending on the type of processing that is taking place, but typically ACH rates across the industry are between 15 and 25 air changes per hour. For RTE product processing facilities, 20–25 ACH is the common industry practice.
I have had experience working with a food company that manufactured dietary supplements, containing a sensitive bacterial probiotic, where the observed ACH was in excess of 400 changes per hour. Companies manufacturing medical foods or foods for prenatal use may operate at even higher rates of around 600 ACH. Similar to the points above regarding filtration, higher ACH rates may require upsizing of HVAC equipment. Higher rates also drive additional energy usage, and when these factors are combined with increased fresh air intake, the energy penalties can be significant. This goes back to the simple fact that achieving food safety is a product- and process-specific activity. What works for one segment of the industry might not work for another.

“While the food safety risks due to surface contamination are understood and the cleaning measures validated, these procedures have some limitations and drawbacks.”
As a simple matter of physics, things suspended in the air will eventually succumb to the effects of gravity and be pushed back down to the earth's surface. The effects of gravity also apply to bacteria and other small particles suspended in the air. This means that airborne bacteria will eventually fall from the air, adding to the existing bioburden and microbiome of surfaces throughout the food processing establishment. This incipient form of contamination affects exposed food and food ingredients, as well as other critical food contact surfaces, including those surfaces with a Zone 1 designation.
The science of surface cleaning and disinfection in the food industry is highly evolved. Cleaning and disinfection methods have been validated and verified as effective. For many decades, detergents, acids, quaternary ammonium compounds (QACs), ozone, halogens, peroxides, and alkali have all been used for these purposes. The typical surface cleaning regimen involves several or all of the steps listed below:12
- Cleaning: Removal of food soil and other residues.
- Disinfection: Inactivation of vegetative bacteria, yeasts, and molds.
- Sanitizing: In the U.S., this means the reduction of microorganisms to safe levels defined by public health authorities; typically a 99.999 percent (5 log10) reduction occurring within 30 seconds for food contact surfaces. For other non-contact surfaces, a 99.9 percent (3 log10) reduction is satisfactory.
- Sterilization: Refers to the statistical destruction and elimination of all living organisms.
While the food safety risks due to surface contamination are understood and the cleaning measures validated, these procedures have some limitations and drawbacks. Many of the chemicals highlighted here are highly reactive, some are toxic, and others are explosive. They may also interact with the environment, workers, and food processing equipment in negative ways and force the facility to shut down during the cleaning and sanitation cycles. Workers on the sanitation crews, due to health and safety concerns, are required to wear special PPE. Certain compounds are very corrosive and can cause serious damage to the food facility, its processing machinery, and ancillary equipment. Moreover, many of these compounds are selective in their biocidal activity. For example, QACs are not very effective against gram-negative bacteria, and they exhibit only minimal lethality against bacterial spores or mold.10 Similarly, the chlorinated compounds lose their germicidal effectiveness in the presence of high concentrations of organic materials. They are also unstable in hot water and suffer diminished biocidal capacity.12
Recent Advances in Ambient Air Hygiene and Surface Cleaning
The science of cleaning and food processing hygiene continues to evolve. Several new and interesting techniques for cleaning and sanitizing food processing equipment and food production facilities have emerged over the past decade. Many of these techniques hold great promise for advancing hygienic capability across a highly diverse food supply network. In particular, hydroxyl radicals offer a unique advantage for improving air hygiene and for more efficient food contact surface disinfection.
Hydroxyl Radicals (OH)
It is well established that OH are the means by which nature keeps the air we breathe and our outdoor environment free of unsafe levels of chemicals and microorganisms. Hydroxyl radical reactive air and surface cleaners have been developed to generate the same concentrations of OH indoors as are found outdoors. Hydroxyl sanitizing devices are designed to generate OH by the UV photolysis of water vapor, and they are intended to run continuously in occupied spaces to minimize unsafe levels of chemicals and microorganisms that may otherwise accumulate.4 Hydroxyl radicals are powerful oxidants and react one million times faster and more effectively than hydrogen peroxide, ozone, or hypochlorite, meaning that very small amounts (high-ppm and low-ppb) are effective and safe.
Hydroxyl radicals are highly reactive with both organic and inorganic matter.13 OH has the highest oxidation potential (2.8 volts)12,14 of any compound (see Table 1) other than elemental fluorine, an attribute that drives its reactions with chemical and biological compounds.
“Each step in the 'rings of defense' must be used consciously and with proper consideration, as it may be obvious what caused the noncompliance, and its resolution may be straightforward, simple, and not costly.”


TABLE 1. Comparison of Oxidation Potential Among Various Biocidal Agents12,14
Once formed, OH has one reactive goal: to regain a hydrogen atom (H) and be restored to its stable structure of water (H2O). This is a highly favored reaction both kinetically and thermodynamically. The energy barrier for H atom abstraction is very low, so reaction is extremely fast—on the order of milliseconds. OH will remove an H atom from essentially every organic moiety it comes into contact with.4 This is precisely what makes hydroxyl radicals such good sanitizing agents, as the chemical constituents of bacterial, viral, and fungal cell walls are rich in H atoms. Once an H atom is removed, the remaining compound bears a radical on a carbon atom, which triggers more chemical reactions and results in the rupture of the phospholipid bilayer and cell death.
Hydroxyl radicals are the quintessential reactive oxygen species. Their oxidation characteristics are non-selective, and they can react with almost all types of substances.3,14 This indiscriminate reaction characteristic is also the source of the radical's potent biocidal effects.
In air cleaning devices, OH can be formed by the UV scission of water vapor to yield OH directly, as shown in Equation 1 below:

Once formed, OH decomposes organic compounds (R-H) by removing an H atom to form an organic radical (R), which in turn reacts within milliseconds with oxygen (O2) to form organic peroxyl radicals (ROO), as shown in Equation 2 below.4 ROO radicals are also powerful oxidants and sanitizing agents and have a significantly longer lifetime than the hydroxyl radical, on the order of 12 minutes.14 They are able to circulate throughout the treatment space, sustaining their antimicrobial effects by radical chain reactions with other organic compounds (R'-H,) resulting in VOC decomposition and pathogen inactivation in air and on surfaces.4,14 Organic oxidants ROO are also excellent surface disinfectants.

Other methods are used to generate different reactive species for this purpose. These include forcing air and air/gas mixtures to flow across and through an electric field to generate ions, which are able to inactivate microorganisms based on published data. Another method, called photocatalytic oxidation (PCO), generates reactive oxygen species by using UV energy > 250 nm to activate semiconductor catalysts like titanium dioxide (TiO2) or zinc oxide (ZnO2) coated onto high-surface-area media. The primary mode of action of PCO devices is based on the ability of the catalysts to adsorb VOCs and microorganisms that are decomposed by the reactive oxygen species generated by irradiating the catalyst. The reactive agents include OH, superoxide, and H2O2, some of which can escape the catalyst surface.15
As noted above, the hydroxyl radical is an especially powerful biocide because of its very high oxidation potential. The literature reports that hydroxyl radicals are effective against a wide array of microorganisms, both vegetative forms and spores. The radical is also effective against viruses and mold.
Published data16 show that airborne gram-positive and gram-negative bacteria are reduced by 3–5 log10 cycles after exposure to the hydroxyl radical for 30–120 minutes. The same report also shows the complete inactivation of airborne SARS-CoV-2 virus after an exposure time of 80 minutes. Surface-bound human pathogens E. coli O157:H7, Salmonella, Listeria, and Staphylococcus aureus showed from 2–4 log10 inactivation in less than or equal to 360 minutes of exposure to OH.
Studies published in the Journal of the Air and Waste Management Association further demonstrate the quantitative formation and reaction of OH with VOCs to produce organic peroxyl (ROO) and oxyl (RO) radicals, which are also powerful oxidants that cleanse and sanitize like OH.4 Peroxyl radicals have a much longer effective lifetime than hydroxyl or oxyl radicals, enabling them to sanitize air and surfaces and decompose VOC throughout the treatment space via radical chain reactions that gradually oxidize the individual carbon atoms and release them as CO2.4,14 These cleansing agents are known to kill bacteria, viruses, and mold, and can also rapidly decompose a broad range of aliphatic and aromatic chemicals.5,8 They are a natural way of safely keeping the air free of harmful levels of chemicals and pathogens.
Part 2 of this series, to be published in the February/March issue, will examine a number of other interventions for food plant decontamination including light-based and reactive oxygen-based technologies, as well as peroxide, chlorine dioxide, ozone, and electrolyzed oxidizing water.
References
- Gutowski, M. and S. Kowalczyk. "A Study of Free Radical Chemistry: Their Role and Pathophysiological Significance." Acta biochimia Polonica (2013). https://www.researchgate.net/publication/236065365_A_study_of_free_radical_chemistry_Their_role_and_pathophysiological_significance.
- Atkinson, R. "Kinetics and Mechanisms of the Gas-Phase Reactions of the Hydroxyl Radical with Organic Compounds Under Atmospheric Conditions." Chemical Reviews 86, No. 1 (February 1986). https://pubs.acs.org/doi/10.1021/cr00071a004.
- Crosley, D.R. "The Measurement of OH and HO2 in the Atmosphere." Journal of the Atmospheric Sciences 52, No. 19 (October 1995): 3299–3314. https://journals.ametsoc.org/view/journals/atsc/52/19/1520-0469_1995_052_3299_tmooah_2_0_co_2.xml.
- Crosley, D.R., C.J. Araps, M. Doyle-Eisele, and J.D. McDonald. "Gas-phase photolytic production of hydroxyl radicals in an ultraviolet purifier for air and surfaces." Journal of the Air & Waste Management Association 67, No. 2 (2017): 231–240. https://www.tandfonline.com/doi/full/10.1080/10962247.2016.1229236.
- Li, M., E. Karu, C. Brenninkmeijer, H. Fischer, J. Lelieveld, and J. Williams. "Tropospheric OH and stratospheric OH and Cl concentrations determined from CH4, CH3Cl, and SF6 measurements." Nature. September 11, 2018. https://www.nature.com/articles/s41612-018-0041-9.
- Graham, D. "In-Plant Air Handling and Food Safety: There is a Connection." Food Safety Magazine. June 1, 2011. https://www.food-safety.com/articles/3832-in-plant-air-handling-and-food-safety-there-is-a-connection.
- Dobeic, M. E. Kenda, J. Micunovic, and I. Zdovc. "Airborne Listeria spp. in the Red Meat Processing Industry." Czech Journal of Food Sciences 29, No. 4 (August 2011): 441–447. https://www.researchgate.net/publication/260157343_Airborne_Listeria_spp_in_the_Red_Meat_Processing_Industry.
- U.S. Food and Drug Administration (FDA). "Leafy Greens STEC Action Plan." Content current as of November 17, 2023. https://www.fda.gov/food/foodborne-pathogens/leafy-greens-stec-action-plan.
- Oliveria, M., B. Tiwari, and G. Duffy. "Emerging Technologies for Aerial Decontamination of Food Storage Environments to Eliminate Microbial Cross-Contamination." Foods 9, No. 12 (2020). https://www.mdpi.com/2304-8158/9/12/1779.
- Moore, G., C. Griffith, and A. Peters. "Bactericidal Properties of Ozone and its Potential Application as a Terminal Disinfectant." Journal of Food Protection 63, No. 8 (August 2000) 1100–1106. https://pubmed.ncbi.nlm.nih.gov/10945587/.
- American Society of Heating, Refrigerating, and Air-Conditioning Engineers (ASHRAE). "ANSI/ASHRAE Standard 62.1: Ventilation for Acceptable Indoor Air Quality." https://www.ashrae.org/File%20Library/About/Government%20Affairs/Advocacy%20Toolkit/Virtual%20Packet/62-1-2016-Fact-Sheet.pdf.
- Centers for Disease Control and Prevention (CDC). "Improving Ventilation in Buildings." May 11, 2023. https://www.cdc.gov/coronavirus/2019-ncov/prevent-getting-sick/improving-ventilation-in-buildings.html.
- Keener, L. "Chapter 28: Improving Hygiene in Food Transportation." Handbook of Hygiene Control in the Food Industry. CRC Press, 2005.
- Khankari, K., C. Araps, and D. Crosley. "CFD Analysis of Hydroxyl Technology to Reduce Risk of Indoor Pathogen Transmission." ASHRAE Journal 66, No. 3 (March 2024): 36–43. https://ansight.com/publications/cfd-analysis-of-hydroxyl-technology-to-reduce-risk-of-indoor-pathogen-transmission/.
- Keener, L. "Hurdling New Technology Challenges: Investing in Process Validation of Novel Technologies." Food Safety Magazine. February 1, 2006. https://www.food-safety.com/articles/4120-hurdling-new-technology-challenges-investing-in-process-validation-of-novel-technologies.
- "Hydroxyl Radical." Topic: Earth and Planetary Sciences. ScienceDirect. https://www.sciencedirect.com/topics/earth-and-planetary-sciences/hydroxyl-radical.
Larry Keener, CFS, PA is President and CEO of International Product Safety Consultants. He is also a member of the Editorial Advisory Board of Food Safety Magazine.