SANITATION
By Larry Keener, CFS, PA, President and CEO, International Product Safety Consultants
Hydroxyl Radicals for a Radical Advantage in Food Processing Hygiene: A Survey of Biocides Used by the Food Industry—Part 2
Biocidal chemicals and other physical/chemical techniques may be used together, in a strategic manner, to achieve an overall improvement in plant hygiene
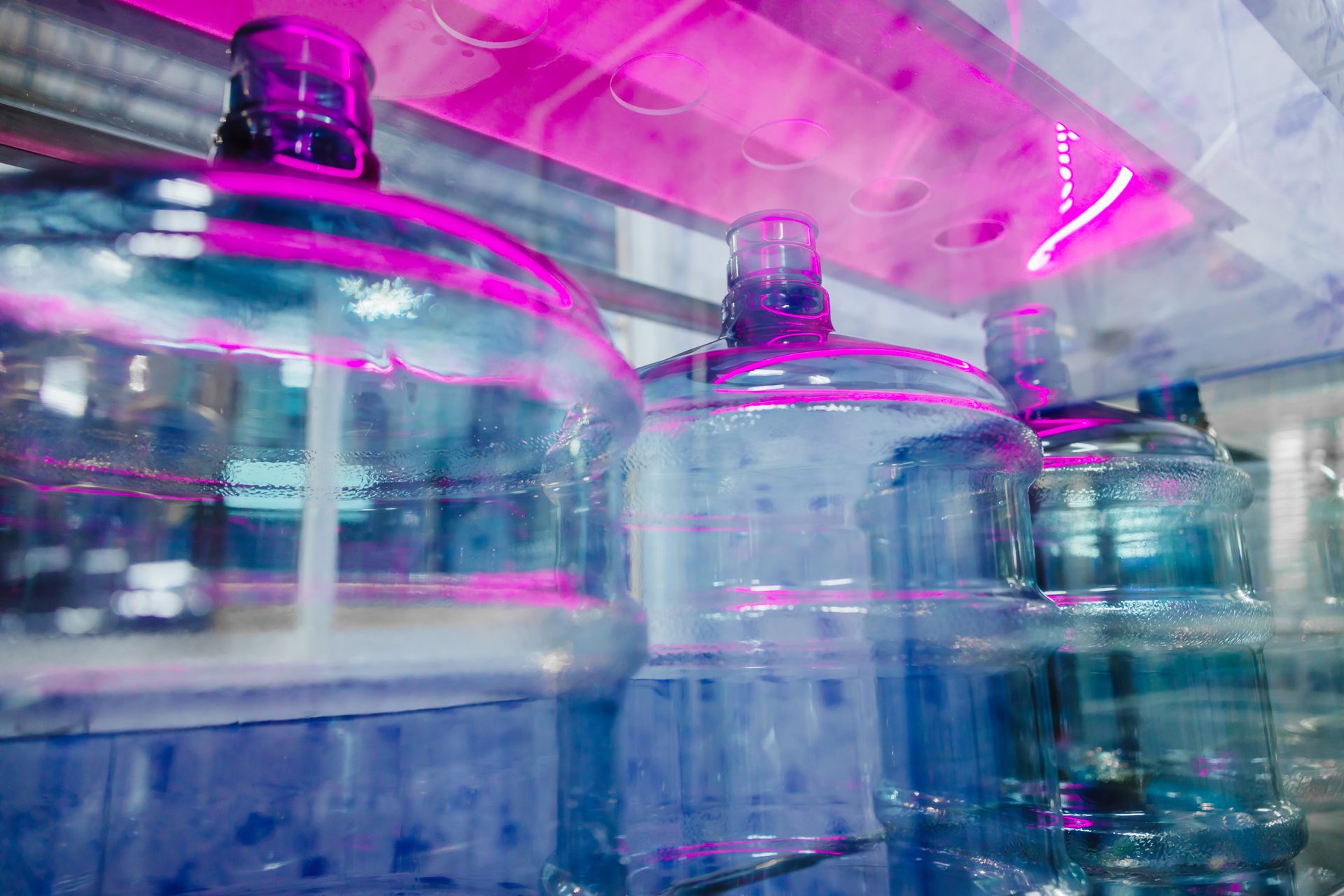
Image credit: coffeekai/iStock/Getty Images Plus via Getty Images
SCROLL DOWN
Part 117 of this article series discussed the benefits of hydroxyl radical reactive air cleaning technology for decontamination of ambient air in food plants. Lab-based and real-world case studies have shown that the use of hydroxyl radicals, at the appropriate concentration, offers a proven, highly effective, safe, and scalable approach to food plant hygiene and food safety.
The conclusion of this series examines a variety of other intervention technologies for decontamination of surfaces and equipment in food facilities, including light-based and reactive oxygen-based technologies, peroxide, chlorine dioxide, ozone, and electrolyzed oxidizing water (EOW).
Light-Based Interventions Using UV, UVC, and UVC-LED
Over the past 30 years, a number of researchers have investigated pulsed light and other light-based technologies as a means for decontaminating food and food contact surfaces, non-opaque liquids, and food packaging materials. For the light-based technologies most often used by the food and beverage industries, three ultraviolet (UV) wavelength sub-divisions are widely reported in the scientific literature:
- UVA (315–400 nanometers, or nm)
- UVB (280–315 nm)
- UVC (< 280 nm).
Each UV wavelength range has specific effects on biological materials. UVC is frequently referred to as the "germicidal wavelength" and is centered at 254 nm.
"Pulsed light" refers to broad-spectrum radiation delivered as intense, intermittent, short-duration pulses. A typical pulsed light lamp produces polychromatic radiation in the wavelength range of 100–1,100 nm,18 although the wavelength range can vary based on the lamp source. Pulsed light is also referred to as high-intensity light, broad-spectrum white light, intense pulsed light, pulsed white light, and pulsed UV light.18,19,20 In general, the majority of energy from pulsed light comes from the UV portion of the spectrum.18,20,21 The wavelengths generated by this method are hazardous to humans, particularly the eyes, and care must be taken to prevent exposure during use.
Pulsed light is effective for sterilization of surfaces and highly transparent liquids, such as water, and has been successfully used to inactivate bacteria, molds, spores, and viruses in various food materials and on food contact surfaces.18,19,20 Pulsed light is an effective tool for surface decontamination of packaging materials and food contact surfaces.
In the mid-1990s, the author worked with a team of scientists to validate, commission, and install a high-intensity pulsed light system in a commercial food establishment in the U.S. The system was used for decontaminating the contact surface of a primary food package to eliminate vegetative pathogens and spoilage flora. The pulsed light process was validated using the spores of Bacillus subtilis A. In this instance, the traditional disinfecting biocide, H2O2, was deemed incompatible because its residues would have remained on the packaging material and resulted in oxidative rancidity in the finished product. The line speed used by the process did not allow time for the complete disassociation of H2O2 to oxygen and water, prior to the introduction of the food into the treated container.
Recently, Haughton et al.22 studied the efficacy of pulsed light for inactivation of microorganisms on packaging surfaces. A 5-second pulsed light treatment of packaging materials and food contact surfaces resulted in 3.56-, 4.69-, and 4.60-log10 CFU/cm2 reductions in the populations of Campylobacter jejuni, Escherichia coli, and Salmonella enteritidis, respectively.
UV treatment has some advantages over chemical sanitizers, as no chemicals are added to or remain in the production environment. UV systems are easy to install and relatively maintenance-free, have low capital costs, and avoid chemical storage hazards. Biocidal action requires direct exposure to treated surfaces and sufficient exposure time on the order of 5–10 minutes to be effective. Areas that are shadowed are not treated. There are safety issues associated with exposure to UV radiation, as it is hazardous if it comes in contact with living organisms, particularly the skin and eyes.
Maclean et al.23 investigated the use of light-emitting diodes (LEDs) with a specific wavelength of 405 nm. This work was performed to reduce methicillin-resistant Staphylococcus aureus (MRSA) and other important human pathogens. Both gram-positive and gram-negative bacteria were effectively reduced, and MRSA was completely eliminated even at high concentrations.23 The authors concluded that this technology needs further development for commercial applications. However, the use of UVC-LED is a promising and much investigated technique to provide effective air decontamination in both clinical and nonclinical facilities.23 Blue light LEDs (405 nm) have recently emerged in research and are of great interest because of their apparent bactericidal properties. In order for this method to be incorporated within existing air handling systems used in food production facilities, studies are required to demonstrate efficacy and scalability.

“The use of gaseous sanitizers as fogging agents has provided improved antimicrobial capacity over liquid disinfectants because they are more spreadable, uniform, and can reach difficult or hidden areas.”
Reactive Oxygen-Based Technologies
Fogging using various oxidizing agents—such as chlorine dioxide (ClO2), organic acids, hydrogen peroxide (H2O2), and ethanol with a demonstrated antimicrobial activity—have been considered as suitable contact surface sanitizers.6,9,21 The use of gaseous sanitizers as fogging agents has provided improved antimicrobial capacity over liquid disinfectants because they are more spreadable, uniform, and can reach difficult or hidden areas.6,9
Fogging involves the dispersal of finely disposed droplets of a disinfectant and is applied after cleaning to guarantee that all surfaces and equipment in the food processing plant have received the sanitizer. To date, it is reported that relatively little research has been carried out regarding the use of this fogging technique in food processing plants or on food processing machines and equipment. Holah et al.6,9 found that fogging was less effective as compared to other decontamination methods, such as the use of ozone or UV radiation. Burfoot et al.6 showed that fogging was an effective means to reduce bacterial counts on upward-facing surfaces, but not on vertical or downward-facing surfaces. Burfoot et al.6 also concluded that fogging can reduce airborne microorganism levels, but is dependent on fogging drop size and dispersion patterns. Broadcast fogging has the associated risk of incidental contamination of exposed foods in the work environment.
Peroxides
Hydrogen peroxide (H2O2) generates OH free radicals in aqueous solutions containing the ferrous ion (Fe+2), which acts as a catalyst. Such solutions serve as a highly active biocide since OH radicals react with the lipids, carbohydrates, and proteins that constitute pathogen cell walls, causing structural changes that result in leakage of cellular content and cell death. Once breached, OH can damage cellular DNA, preventing reproduction and contributing to cell death.6,21 H2O2 and OH solutions have a wide range of activity on vegetative cells of bacteria, as well as on spores (bacterial and fungi) and viruses, but less activity against catalase-positive microorganisms.21 The enzyme catalase is used commercially to inactivate H2O2.
Hydrogen peroxide is used as an oxidizer, bleaching agent, and antiseptic, usually as a dilute solution (3–6 percent by weight) in water for consumer use and in higher concentrations (33 percent) for industrial use. It decomposes slowly into water and elemental oxygen when exposed to light, and rapidly in the presence of organic or reactive compounds. Under the right conditions, it leaves little to no residue. It can be used in the vapor phase, often in combination with heat, since high temperature increases its antimicrobial ability.6 Based on the author's own research working with hot (100 °F/37.8 °C) H2O2 at a concentration of 33 percent, it was shown that spores of Bacillus subtilis A were reduced by greater than 5 log10 after an exposure time of less than 1 minute. A number of aseptic packaging machines use H2O2 in spray form as a sterilant for treating both packaging material and the sterile zone of the aseptic filling machine.25 Hydrogen peroxide vapor technology has been used primarily in the medical, biological, and pharmaceutical industries, but is commercially available and has demonstrated capacity to decontaminate large, unoccupied spaces.
Peroxyacetic acid, also known as peracetic acid (PAA), a mixture of hydrogen peroxide and acetic acid, is used extensively by the food industry as a sanitizing agent. PAA is effective against both gram-positive and gram-negative bacteria.9,21 Its mode of action has not been fully elucidated; however, it is generally accepted that it derives its germicidal power by disrupting cell wall integrity. PAA has also been shown to be effective in the removal of biofilms attacking both the planktonic and sessile bacteria that form the film. PAA is highly corrosive and unstable in concentrated form and is on the U.S. Environmental Protection Agency's Hazardous Substances List.9
Chlorine Dioxide
Chlorine dioxide (ClO2) is another strong oxidizing agent that was developed for water disinfection applications. Its disinfection activity is less influenced by pH and results in less harmful byproducts than traditional chlorine treatment.9 Chlorine dioxide gas has equal or greater antimicrobial potency than chlorine. Its antimicrobial activity is primarily due to the oxidation of cell-surface proteins, which destabilize the structure of cell membranes.6,9,21 Due to its high oxidative capacity (5 electrons), the required amount of ClO2 is lower, and the required contact time shorter, to obtain the same antimicrobial effect as chlorine.6 The combination of a relatively low oxidation potential and high oxidative capacity means that chlorine dioxide is an effective biocide with little or no corrosive effects.6,9 Chlorine dioxide has been effectively used for environmental decontamination, particularly within food processing environments.6,9,21 Although gaseous or liquid systems are widely used, there is little information on the delivery of this sanitizer as a biocide for air decontamination. There are limits to the practical application of chlorine dioxide, as it is toxic to humans at concentrations greater than 1,000 ppm.6 Furthermore, chlorine dioxide gas must be generated onsite, because it cannot be compressed and stored or transported under pressure.6
In solution, various chlorine-based compounds form hypochlorous acid (HOCl).9 HOCl is a strong oxidizing agent (1.49 volts), but not as strong as H2O2, which has an oxidation potential of 1.8 volts.9 HOCL exerts its germicidal effect by attacking bacterial membranes and by inhibiting several other vital metabolic processes. The effectiveness of HOCl is greatly reduced in the presences of high concentrations of soil and other organic materials.21 Again, however, the major disadvantage to chlorine compounds are their corrosiveness and worker health and safety issues.
“Each step in the 'rings of defense' must be used consciously and with proper consideration, as it may be obvious what caused the noncompliance, and its resolution may be straightforward, simple, and not costly.”


TABLE 2. Activity of Biocides Commonly used by the Food Processing Industry
Ozone
Ozone (triatomic oxygen, or O3) is a very reactive allotrope of oxygen (O2). It is used to sanitize and deodorize, either in an aqueous solution or as a gas phase. At the concentrations of gas required to be effective, ozone is toxic and corrosive. At higher concentrations, it is explosive. Ozone is widely used by the food industry as a disinfectant due to its strong oxidizing power.24
Ozone tends to accumulate indoors even under normal HVAC ventilation conditions, as outdoor return air also contains ambient ozone. Ozone's primary loss mode is due to surface deposition. The most common application of ozone is in the disinfection of water, where it can exert a powerful biocidal effect. When ozone is dissolved in water in the presence of various metal salts like Fe+2, it decomposes to form molecular oxygen and other highly reactive free radicals HO2, OH, and H.24 Vegetative cells suspended in water are highly susceptible to ozone. It has been reported that a 6–7 log10 reduction in vegetative bacteria occurred after 5 minutes of exposure to ozone at concentrations in the range of 0.01–1.0 milligrams per liter (mg/L).6,9,24 Ozone is also being tested for use in the disinfection of air.24
Ozone is a very potent biocide with an oxidizing potential of 2.075 volts.9,24 It is more powerful than chlorine, chlorine dioxide, hydrogen peroxide, or peroxyacetic acid.6,9,24 The only oxidant with greater oxidizing power and higher rates of reaction with VOCs and microorganisms is the OH radical, which reacts one million times faster than ozone.4 Nonetheless, ozone is a strong antimicrobial agent with a wide range of activity against bacteria, fungi, spores, protozoa, and viruses.6,9,24 Its effectiveness to kill microorganisms is a function of concentration, temperature, contact time, and the substrate on which the microorganisms reside, as well as of the specific characteristics of the target microorganism. In the case of bacteria, ozone attacks the phospholipid bilayer and other structures of the cell's outer membrane, causing changes in the cell's permeability and, ultimately, death of the cell due to its lysis.9 Viruses in water are also shown to have an extremely low tolerance to ozone.24
Kim and Yousef6 tested ozone against Pseudomonas fluorescens, E. coli O157:H7, Leuconostoc mesenteroides, and L. monocytogenes in water. These researchers have reported that exposure to 2.5 ppm of O3 for 40 seconds resulted in a 5.0–6.0 log10 reduction in these bacteria. They also found that E. coli O157:H7, an important human pathogen, was the most resistant to ozone treatment. Taylor and Chana,6 in their research on the inactivation kinetics (both airborne and surface-bound) of Pseudomonas aeruginosa, have reported a 2.0 log10 reduction in both airborne and surface-adhered organisms in 2 hours when exposed to 2 ppm of ozone. Ozone gas has also been shown to be effective in reducing viable bacteria attached to stainless steel surfaces.10 Laboratory-scale studies conducted in an aerobiology cabinet showed that an exposure of 4 micrograms (µg)/mL of ozone for 5–10 min caused a 2–4 log10 inactivation of airborne P. aeruginosa. Additionally, Cullen and Norton6 reported ozone is an efficient method for inactivating airborne molds. At these concentrations, ozone is toxic to humans, and treatment must be done in unoccupied spaces.
At these and higher concentrations, ozone is used to decompose VOCs and decontaminate and deodorize air in unoccupied spaces. Ozone reacts slowly with most VOCs and yields incomplete organic oxidation byproducts. Little is known about the chemical byproducts left behind by these processes. Ozone can adversely affect indoor plants and damage materials such as rubber, electrical wire coatings, and the myriad materials of construction and fabrication found in a food processing establishment.
Ozone applications in food processing have been legally approved, although to varying degrees, in North America, Australia, New Zealand, Japan, and several European countries.6,24 Ozone was deemed accepted for use by the U.S. Food and Drug Administration (FDA) in 2001 as an antimicrobial agent for use in the processing of human food. FDA has authorized the use of ozone in both its aqueous and gaseous forms. However, ozone and ozone generators are costly, and ozone requires fastidious caution to use or to store, making it poorly suited for tasks that could be accomplished with another oxidizing agent.

“The concept of using electrolyzed oxidizing water for cleaning and disinfection of food facilities and as a biocide in air treatments has existed for some time, but in reality the use of this technique has not caught on as a sanitation tool in the food processing industry.”
Electrolyzed Oxidizing Water
Another interesting application is the use of electrolyzed oxidizing water (EOW) as a cleaner and sanitizer. EOW can be used in acidic, base, or neutral solutions to both clean and sanitize surfaces and ambient air. EOW, containing both NaOH and HOCL, is usually produced by passing a diluted (non-iodized) salt (NaCl) through an electrolytic cell, where the pH of the generated alkaline water is about 11–12, while the pH of acid water is around 1–3.25 It is considered that the alkaline solution (NaOH) exerts a cleaning effect, whereas the acid solution (HOCl) has a strong biocidal activity. EOW can be generated using an electrolytic cell fitted with a membrane that separates the anode from the cathode. Using this method, both acid and alkaline EOW can be produced simultaneously; acid EOW is produced at the anode, and alkaline EOW is formed at the cathode. At the anode, the primary end products are hypochlorous acid (HOCl), hydrochloric acid (HCl), and hypochlorite ions (OCl-). At the cathode, the electrolytic products are hydrogen gas (H2) and sodium hydroxide (NaOH).25 Neutral EOW is produced in an electrolytic cell that has no membrane separation between the anode and cathode, or by mixing acid EOW with alkaline EOW.
EOW has been applied in food production as a spray or used for broadcast fogging.25 Cold fogging with electrolyzed water has been effective for decontaminating rooms, mainly in healthcare environments.6 A treatment with electrolyzed water applied by fogging was able to reduce MRSA and Acinetobacter baumannii.6 Electrolyzed water has been evaluated for several food safety industrial applications, including use as a fresh produce wash,6 cleaning and decontamination of dairy facilities,6 food processing applications, and pig and poultry applications.6,25 EOW has also been used in the food and beverage industry for cleaning and decontaminating glass bottles and jars.
The concept of using EOW for cleaning and disinfection of food facilities and as a biocide in air treatments has existed for some time, but in reality the use of this technique has not caught on as a sanitation tool in the food processing industry.25 A review of the literature on the subject confirms that its use (in acid form) as a biocide might cause damage to food processing equipment and harm the health of workers who might be exposed when EOW is used as a broadcast fogging agent.25 Another important consideration that needs to be addressed when using this material as a fogging agent is the possibility of it becoming an indirect food additive. The addition of EOW (NAOH and HOCl) to food during processing might run counter to FDA regulations. Hence, when EOW is used as either a spray or a fog, all exposed food and food ingredients would likely need to be removed from the line, requiring a temporary production shutdown.
Takeaway
The three most frequently used methods for indoor air purification, in order of effectiveness, are:
- Source control: Eliminate or control the sources of pollution
- Ventilation: Dilute and exhaust pollutants through outdoor air ventilation
- Air cleaning: Remove pollutants through proven air cleaning methods.
Of the three, the first approach—source control—is the most effective. This involves minimizing the use of products and materials that cause indoor pollution, employing good hygiene practices to minimize biological contaminants (including the control of humidity and moisture, and occasional cleaning and disinfection of wet or moist surfaces), and using good housekeeping practices to control particles.
While it is true that source control is the most effective of the available options, it is also true that in food processing and production operations, this might also be the most difficult to achieve. The very nature of the business generates sources of indoor pollution. Moisture, steam, dust particles, aerosols, and condensate are often the byproducts of food processing. Certain industry segments produce more of these pollutants than others. For example, the airborne biological load within a pig processing facility will likely be exceedingly high for both organic matter and a plethora of different kinds of microorganisms, including many human pathogens. In this example, air cleaning and ventilation are likely better sanitizing options.
The extreme example of the swine slaughtering factory aside, in general, food processing operations create environmental conditions that are favorable for sustaining microorganisms—both surface-bound and airborne. Raw materials, people, and the machinery used in those operations are the primary sources of contamination. As previously reported, the food safety literature is replete with credible scientific papers confirming air as a vector in the dissemination of microorganisms.

“OH air purification systems have been proven to be safe and scalable to hundreds of thousands of cubic feet of space, with very high efficacy in minimizing pathogen loads and decomposing VOCs. They are also highly effective at minimizing odors.”
It is clear that there is no panacea for mitigating the pernicious effects of microbes in food processing facilities. It is possible, however, to reduce the risk of microbial contamination of food products using an integrative approach to achieve and sustain high levels of hygiene in such environments. Biocidal chemicals and other physical/chemical techniques discussed in this article may be used together, in a strategic manner, to achieve an overall improvement in plant hygiene.
This strategy must consider the potential for dangerous reactions occurring when certain of the compounds are mixed. For example, chlorinated compounds should never be mixed with ammonia due to the potential for liberating dangerous chloramine gases. Other biocides may be used sequentially and without great risk of explosion or harm to workers. Using sodium hydroxide as a cleaner, followed by a water rinse and then hypochlorous acid as a disinfectant, is a common practice in the industry.
Aside from the issue of incompatibility among the biocides, there are also the issues of corrosion and damage to the food processing equipment. These problems are associated primarily with chemical biocides. Other techniques, such as UV light-based technologies, do not suffer these side effects but are limited by other factors, such as shadowing and the need to be used in unoccupied spaces. Another consideration when using biocides contained in water is the fact that they contribute additional moisture in the factory environment that favors microbial growth.
Air purification systems that generate hydroxyl radicals can be used continuously and effectively in conjunction with strategies 1 and 2—source control and ventilation. OH air purification systems have been proven to be safe and scalable to hundreds of thousands of cubic feet of space, with very high efficacy in minimizing pathogen loads and decomposing VOCs. They are also highly effective at minimizing odors. In addition, out of the various biocide solutions discussed above, the use of hydroxyl radicals is the only solution that is safe for use around people, animals, and plants. Some hydroxyl systems are designed to generate the same steady-state concentration of OH found outdoors and can be used continuously, since OH does not accumulate; it reacts in less than a second with airborne pathogens and VOCs. Byproducts are also decomposed rapidly, yielding CO2 and H2O, as is the case outdoors. The generation of hydroxyl radicals requires only UV energy and does not use any chemical agents; therefore, it does not pose any risk to the food itself. Lastly, hydroxyl radicals do not have an adverse impact on equipment, materials, or electronics. It is important to ensure that the system selected can generate the required steady-state concentration of hydroxyl radicals for the size and potential contaminant load of the environment.
As reported, hydroxyl generators can be integrated into a facility's HVAC and air handling systems, thereby ensuring that clean, hygienic air will replace fouled air in a continuous cycle. This cleansing effect can be further enhanced by manipulating the number of air changes per hour or by manipulating the sensitivity of the final filter in the HVAC system. Using this approach will have a positive impact on surface-bound microorganisms, as well. The OH radical, along with the ROO "organic oxidant" radical, collectively clean the air and attack surface-bound microbial contaminants and biofilms in a continuous manner. Reducing the numbers of viable microbial propagules in the air will result in fewer microorganisms on surfaces facility-wide. Using hydroxyl sanitizing systems in concert with established cleaning methods enables a shortening of the cleaning and sanitation cycles. Fewer organisms on food contact surfaces will require less time for their removal, thereby creating greater opportunity to use the facility's production assets for their intended purpose—the manufacture of safe food products. Even small gains in productivity have the potential for large gains in profits. This is precisely the radical advantage of using OH radicals in a comprehensive, integrative approach to food facility hygiene.
References
- Gutowski, M. and S. Kowalczyk. "A Study of Free Radical Chemistry: Their Role and Pathophysiological Significance." Acta biochimia Polonica (2013). https://www.researchgate.net/publication/236065365_A_study_of_free_radical_chemistry_Their_role_and_pathophysiological_significance.
- Atkinson, R. "Kinetics and Mechanisms of the Gas-Phase Reactions of the Hydroxyl Radical with Organic Compounds Under Atmospheric Conditions." Chemical Reviews 86, No. 1 (February 1986). https://pubs.acs.org/doi/10.1021/cr00071a004.
- Crosley, D.R. "The Measurement of OH and HO2 in the Atmosphere." Journal of the Atmospheric Sciences 52, No. 19 (October 1995): 3299–3314. https://journals.ametsoc.org/view/journals/atsc/52/19/1520-0469_1995_052_3299_tmooah_2_0_co_2.xml.
- Crosley, D.R., C.J. Araps, M. Doyle-Eisele, and J.D. McDonald. "Gas-phase photolytic production of hydroxyl radicals in an ultraviolet purifier for air and surfaces." Journal of the Air & Waste Management Association 67, No. 2 (2017): 231–240. https://www.tandfonline.com/doi/full/10.1080/10962247.2016.1229236.
- Li, M., E. Karu, C. Brenninkmeijer, H. Fischer, J. Lelieveld, and J. Williams. "Tropospheric OH and stratospheric OH and Cl concentrations determined from CH4, CH3Cl, and SF6 measurements." Nature. September 11, 2018. https://www.nature.com/articles/s41612-018-0041-9.
- Graham, D. "In-Plant Air Handling and Food Safety: There is a Connection." Food Safety Magazine. June 1, 2011. https://www.food-safety.com/articles/3832-in-plant-air-handling-and-food-safety-there-is-a-connection.
- Dobeic, M. E. Kenda, J. Micunovic, and I. Zdovc. "Airborne Listeria spp. in the Red Meat Processing Industry." Czech Journal of Food Sciences 29, No. 4 (August 2011): 441–447. https://www.researchgate.net/publication/260157343_Airborne_Listeria_spp_in_the_Red_Meat_Processing_Industry.
- U.S. Food and Drug Administration (FDA). "Leafy Greens STEC Action Plan." Content current as of November 17, 2023. https://www.fda.gov/food/foodborne-pathogens/leafy-greens-stec-action-plan.
- Oliveria, M., B. Tiwari, and G. Duffy. "Emerging Technologies for Aerial Decontamination of Food Storage Environments to Eliminate Microbial Cross-Contamination." Foods 9, No. 12 (2020). https://www.mdpi.com/2304-8158/9/12/1779.
- Moore, G., C. Griffith, and A. Peters. "Bactericidal Properties of Ozone and its Potential Application as a Terminal Disinfectant." Journal of Food Protection 63, No. 8 (August 2000) 1100–1106. https://pubmed.ncbi.nlm.nih.gov/10945587/.
- American Society of Heating, Refrigerating, and Air-Conditioning Engineers (ASHRAE). "ANSI/ASHRAE Standard 62.1: Ventilation for Acceptable Indoor Air Quality." https://www.ashrae.org/File%20Library/About/Government%20Affairs/Advocacy%20Toolkit/Virtual%20Packet/62-1-2016-Fact-Sheet.pdf.
- Centers for Disease Control and Prevention (CDC). "About Ventilation and Respiratory Viruses." May 11, 2023. https://www.cdc.gov/coronavirus/2019-ncov/prevent-getting-sick/improving-ventilation-in-buildings.html.
- Keener, L. "Chapter 28: Improving Hygiene in Food Transportation." Handbook of Hygiene Control in the Food Industry. CRC Press, 2005.
- Khankari, K., C. Araps, and D. Crosley. "CFD Analysis of Hydroxyl Technology to Reduce Risk of Indoor Pathogen Transmission." ASHRAE Journal 66, No. 3 (March 2024): 36–43. https://ansight.com/publications/cfd-analysis-of-hydroxyl-technology-to-reduce-risk-of-indoor-pathogen-transmission/.
- Keener, L. "Hurdling New Technology Challenges: Investing in Process Validation of Novel Technologies." Food Safety Magazine. February 1, 2006. https://www.food-safety.com/articles/4120-hurdling-new-technology-challenges-investing-in-process-validation-of-novel-technologies.
- "Hydroxyl Radical." Topic: Earth and Planetary Sciences. ScienceDirect. https://www.sciencedirect.com/topics/earth-and-planetary-sciences/hydroxyl-radical.
- Keener, L. "Hydroxyl Radicals for a Radical Advantage in Food Processing Hygiene: A Survey of Biocides Used by the Food Industry—Part 1." Food Safety Magazine December 2024/January 2025. https://www.food-safety.com/articles/10017-hydroxyl-radicals-for-a-radical-advantage-in-food-processing-hygiene-a-survey-of-biocides-used-by-the-food-industrypart-1.
- Keener, L. and K. Krishnamurthy. "Shedding Light on Food Safety: Applications of Pulsed Light Processing." Food Safety Magazine. June 4, 2014. https://www.food-safety.com/articles/4310-shedding-light-on-food-safety-applications-of-pulsed-light-processing.
- "Pulsed Light Technology Helps Kill Harmful Food Pathogens." PennState College of Agricultural Sciences. March 30, 2023. https://agsci.psu.edu/magazine/articles/2023/winter-spring/pulsed-light-technology-helps-kill-harmful-food-pathogens.
- Eisenlöffel, L., T. Reutter, M. Horn, S. Schlegal, U. Truyen, and S. Speck. "Impact of UVC-Sustained Recirculating Air Filtration on Airborne Bacteria and Dust in a Pig Facility." PLoS One 14, No. 11 (November 2019). https://pmc.ncbi.nlm.nih.gov/articles/PMC6837447/.
- Huc, J.-F. and L. Keener. "Air Treatment for Improving Food Safety and Gaining Business Advantage." Food Safety Magazine webinar with Pyure. June 12, 2024. https://www.food-safety.com/events/6312-air-treatment-for-improving-food-safety-and-gaining-business-advantage.
- Haughton, P.N., J.G. Lyng, D.J. Morgan, D.A. Cronin, S. Fanning, and P. Whyte. "Efficacy of High-Intensity Pulsed Light for the Microbiological Decontamination of Chicken, Associated Packaging, and Contact Surfaces." Foodborne Pathogens and Disease 8, No. 1 (January 2011): 109–117. https://pubmed.ncbi.nlm.nih.gov/20932088/.
- Maclean, M., S.J. MacGregor, J.G. Anderson, and G. Woolsey. "Inactivation of Bacterial Pathogens Following Exposure to Light From a 405-Nanometer Light-Emitting Diode Array." Applied Environmental Microbiology 75, No. 7 (April 2009): 1932–1937. https://pubmed.ncbi.nlm.nih.gov/19201962/.
- Keener, L. and J. Bricher. "Innovations in Technology: Promising Food Safety Technologies." Food Safety Magazine. April 1, 2007. https://www.food-safety.com/articles/4609-innovations-in-technology-promising-food-safety-technologies.
- Iram, A., X. Wang, and A. Demirci. "Electrolyzed Oxidizing Water and Its Applications as Sanitation and Cleaning Agent." Food Engineering Reviews 13, No. 2 (February 2021): 411–427. https://www.ncbi.nlm.nih.gov/pmc/articles/PMC7893620/.
Larry Keener, CFS, PA is President and CEO of International Product Safety Consultants. He is also a member of the Editorial Advisory Board of Food Safety Magazine.