TESTING
By Jeffrey L. Kornacki, Ph.D., President, Kornacki Microbiology Solutions Inc.
Advancing Food Safety Through an Enhanced Approach to Environmental Monitoring and Source Tracking
Nuanced approaches are needed for industry, academia, and government to reduce contaminants in RTE foods
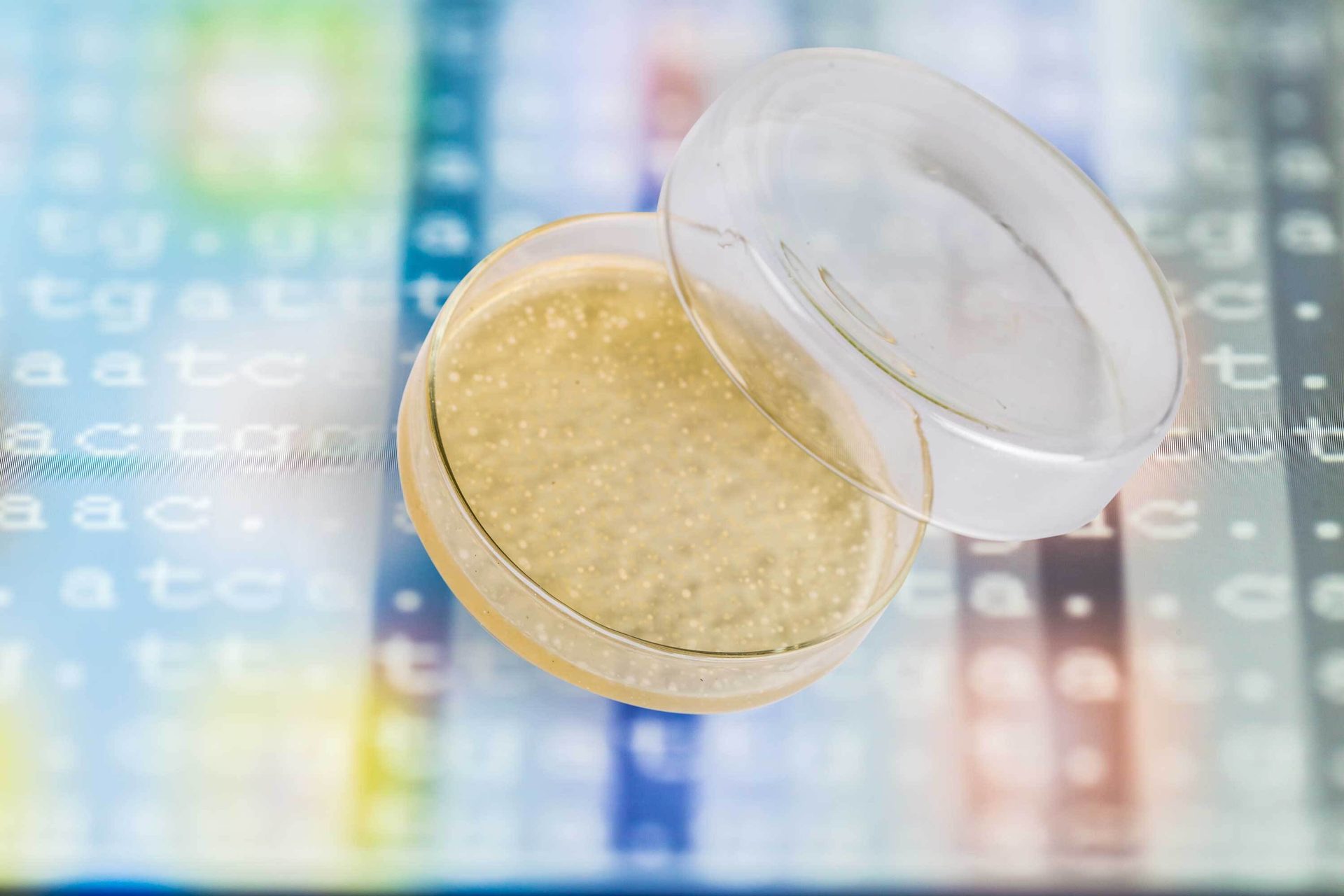
Image credit: jxfzsy/iStock/Getty Images Plus via Getty Images
SCROLL DOWN
The U.S. Centers for Disease Control and Prevention (CDC) has indicated that there are an estimated 38.4 million episodes of domestically acquired foodborne illness from unspecified agents, resulting in 71,878 hospitalizations and 1,686 deaths.1 Thus, there remains a compelling need to enhance approaches to prevent and mitigate contamination of food in the U.S.
In the interest of public health, and in light of scientific papers and perceived government and industry perspectives, this article provides recommendations for enhancements of environmental monitoring approaches, as well as technologies, to improve surveillance actions and the role of whole genome sequencing (WGS) by stakeholders. Classical approaches to environmental monitoring through "seek and destroy," while useful, can be enhanced with source tracking approaches applied in food production facilities. Such approaches have great potential to enhance food safety. However, these methods will look different when applied by academia and government, in contrast to the approaches recommended for industry in this article.
The environment is one of the most important sources of contamination of ready-to-eat (RTE) processed foods2,3,4 in North America. In the author's opinion, based on many hundreds of processing plant visits, it is the most important source of post-lethality contamination of food. However, there is little guidance and much confusion related to the development of an effective environmental monitoring program (EMP). Not surprisingly, there is wide variability in the robustness of such programs in the food industry.
Questions that arise across the industry include the following:
- Why do I need an EMP?
- What might a good EMP look like?
- How might I achieve an EMP that is "best in class?"
- For what organisms or group of organisms should I test?
- How do I determine the number and frequency of these tests?
- What is the role of indicator organism testing in an EMP?
- What is vectoring, and what should be its goal?
- Where does source tracking fit into an EMP?
- What would source tracking look like in a routine program?
- How do I effectively map and manage such a program?
- How can industry, government, and academia get ahead of the next outbreak?
The introduction of WGS to regulatory and industry laboratories has resulted in not only increased detection of disease outbreaks, but also increased recalls, and will very likely result in far more recalls, even as actual illnesses attributable to food may be reduced.5,6 There also remains an urgent need to enhance the food industry's implementation of its EMP plans, as well as academic and regulatory surveillance of foods for "emerging" pathogens.
A Time for Industry Action
Now is the time for the food industry, and especially the low-moisture sector, to ensure that it has nothing less than world-class food safety systems. These systems will encompass many things, including, but not limited to:
- Supplier controls
- Pre-operational swabbing programs for verification of cleaning and sanitization
- Rigorous environmental sampling and corrective action programs
- Appropriate lot-based testing
- Segregation of processing lines
- Hygienic equipment design and repair practices
- Preventive maintenance programs
- Ongoing training and appropriate sanitization approaches.
The need for world-class food safety systems is especially great, given that traditional wet cleaning approaches have been shown to pose greater risk than dry cleaning approaches—particularly when applied in dry food processing environments. While this is a generally understood concept, this article explores a step further.
“Given CDC's estimate that 80 percent of estimated foodborne illnesses occur from unspecified agents, it seems likely that other etiological agents of foodborne illnesses will be discovered.”

Getting Ahead of the Illness Curve
Correlations between environmental contamination and the food processing environment are well known. As early as 1988, Gabis and Faust7 indicated that the likelihood of food contamination from the environment was based on at least six variables:
- Proximity of microbial growth niches to the product stream
- Number of niches in the food processing facility
- Spatial relationships of niches and product stream
- Microbial population in niches
- Degree of niche disruption during operations
- Exposure of the product stream to the environment.
Later, Tompkin et al.8 showed correlations of environmental contamination from floors to packaging lines (Figure 1).
FIGURE 1. Correlation of Percentage of Listeria spp. Isolated From Packaging Lines and Floors to RTE Meat9
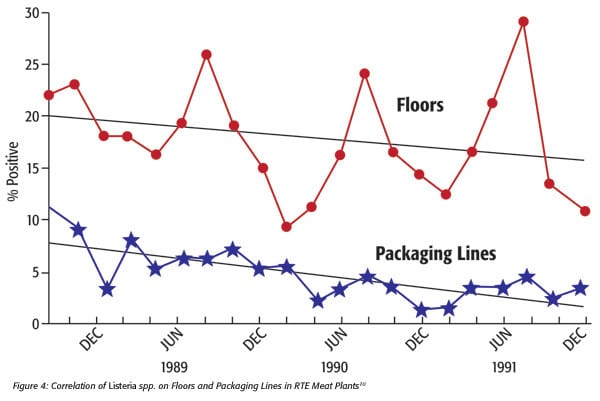
TABLE 1. Pathogens Previously Unrecognized by Date11
More recently, the number of virulent Shiga toxin-producing E. coli (STEC) has dramatically increased to well over 100 O-groups.12
Given CDC's estimate that 80 percent of estimated foodborne illnesses occur from unspecified agents,1 it seems likely that other etiological agents of foodborne illnesses will be discovered. Consider, however: What if we could better identify these agents ahead of outbreaks? What if we had systems in our food production facilities to determine the routes of transmission of these organisms in the production environment and the food? This article addresses both of these considerations.
The author well remembers when Listeria monocytogenes "emerged" in the 1980s as a foodborne pathogen, having been previously regarded as a veterinary pathogen. Now, it is found in nearly all food production facilities.13 What if, back then, we had the tools that we have now? How many outbreaks and recalls could have been prevented if we knew that L. monocytogenes was common in food production facilities? Despite this knowledge, we are still not fully employing the available tools.
Every food producer is required to have an EMP for the organism of greatest risk. The Food Safety Preventive Controls Alliance PCQI manual (FSPCA.2016) states, "An effective environmental monitoring program diligently tries to find the pathogen or indicator of concern so that corrections can be made before product is compromised."14 However, the food industry is largely focused on only two to three pathogens: Salmonella, L. monocytogenes, and in some cases, Shiga toxin-producing strains of E. coli. There are likely other organisms, both known and unknown, for which the question can be asked, "Why wait to monitor and control these, where appropriate?"
The food industry and society, in general, would benefit from understanding the microbiome of food processing environments. Consider, for example, environments that are cold and wet. Such environments are conducive to the growth and survival of cold-tolerating pathogens. Most manufacturers of low-temperature, high-moisture foods monitor their plant environments for Listeria spp. However, other known pathogens can grow and persist in cold, wet environments. Yersinia enterocolitica and Aeromonas hydrophila contain pathogenic strains that are psychrotrophic, as well as many others.15 Might Y. enterocolitica or A. hydrophila be present in such environments? What if their growth niches outnumber Listeria niches in such plants? Should the industry not monitor and control these organisms and at least test for appropriate indicators, with a source tracking paradigm, in such cases? Yet few, if any, food processors monitor for these organisms because their incidence is largely undetermined in their plants. For example, an outbreak of Y. enterocolitica is known to have occurred from pasteurized milk16,17 and has been found in the environments of dairy processing plants.18 Other foodborne illnesses have occurred from this organism, as well, as shown in Figure 2.
FIGURE 2. Estimated Annual Foodborne Disease From Selected Bacterial Pathogens in the U.S. (Source: Centers for Disease Control and Prevention, courtesy of Arthur Liang, M.D., M.P.H.)
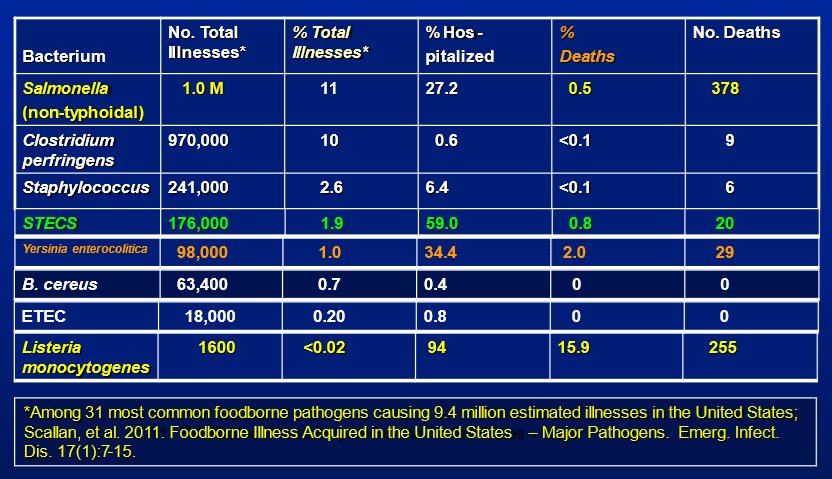
Note, in Figure 2, that Yersinia deaths exceeded STEC deaths.
For the first time in human history, through collaboration with industry, academia, and government, we can develop programs to control microbes that are less surveilled but potentially more common than previously thought. We can also design programs to control microbes that are not yet commonly identified but have been found to cause foodborne illness. This technique involves determination of the microbiome of the environment of food production facilities. This can be done by a variety of techniques, such as 16S ribosomal sequencing, shotgun metagenomics, or others, and then applying the knowledge of virulence factors (where possible) that may be derived from allelic-based WGS (e.g., whole genome multilocus sequence typing, wgMLST) or other approaches.19 Furthermore, through source tracking with appropriate indicators, industry can ask questions about the "how" and "why" of movement of previously ignored microbes or likely emerging pathogens within food production environments.
Source Tracking and "Seek-and-Destroy" Approaches
Source tracking is used by industry and governments alike. In the governmental context, it is for the purpose of establishing probable links among clinical strains, food plant environmental strains, and product strains to one or more of the others listed. Regulatory focus is typically from a broad perspective—e.g., company A may have contaminated a food product and needs to initiate a recall. Historically, various techniques have been used by government and industry in the context of source tracking of bacterial strains.
The industrial perspective is typically at a more granular level and can be represented by seeking to answer the three different questions that follow:
- How did my environment become contaminated (e.g., roof leak, negative air pressure, employee ingress, HVAC system design flaws, poorly designed drains promoting bioaerosol liberation in the plant, improper drain to sump connections, etc.)? This is typically a Zone 3 and 4 investigation.
- Once the microorganism entered the plant, where did it became established, and how did it get there (e.g., foot and wheeled vehicle traffic, bioaerosol movement from high to low pressure areas, overhead structures, sandwiched areas of equipment, etc.)? This is typically a Zone 2 through Zone 4 investigation.
- How did the microorganism get from the environment or ingredient to my finished product? This is typically a Zone 1 and Zone 2 investigation and/or a statistical sampling investigation, in the case of a post-lethality ingredient.
Industry source tracking of isolates also differs from the regulatory perspective, as a company may wish to understand the movement of indicators or pathogens within its processing environment, or in received ingredients, to avoid incurring unnecessary threats to its business.
Source Tracking Approaches
The evolution of source tracking technologies is shown in Figure 3.
FIGURE 3. Trends of "Fingerprinting" for Bacteria (Source: Centers for Disease Control and Prevention, courtesy of Arthur Liang, M.D., M.P.H.)
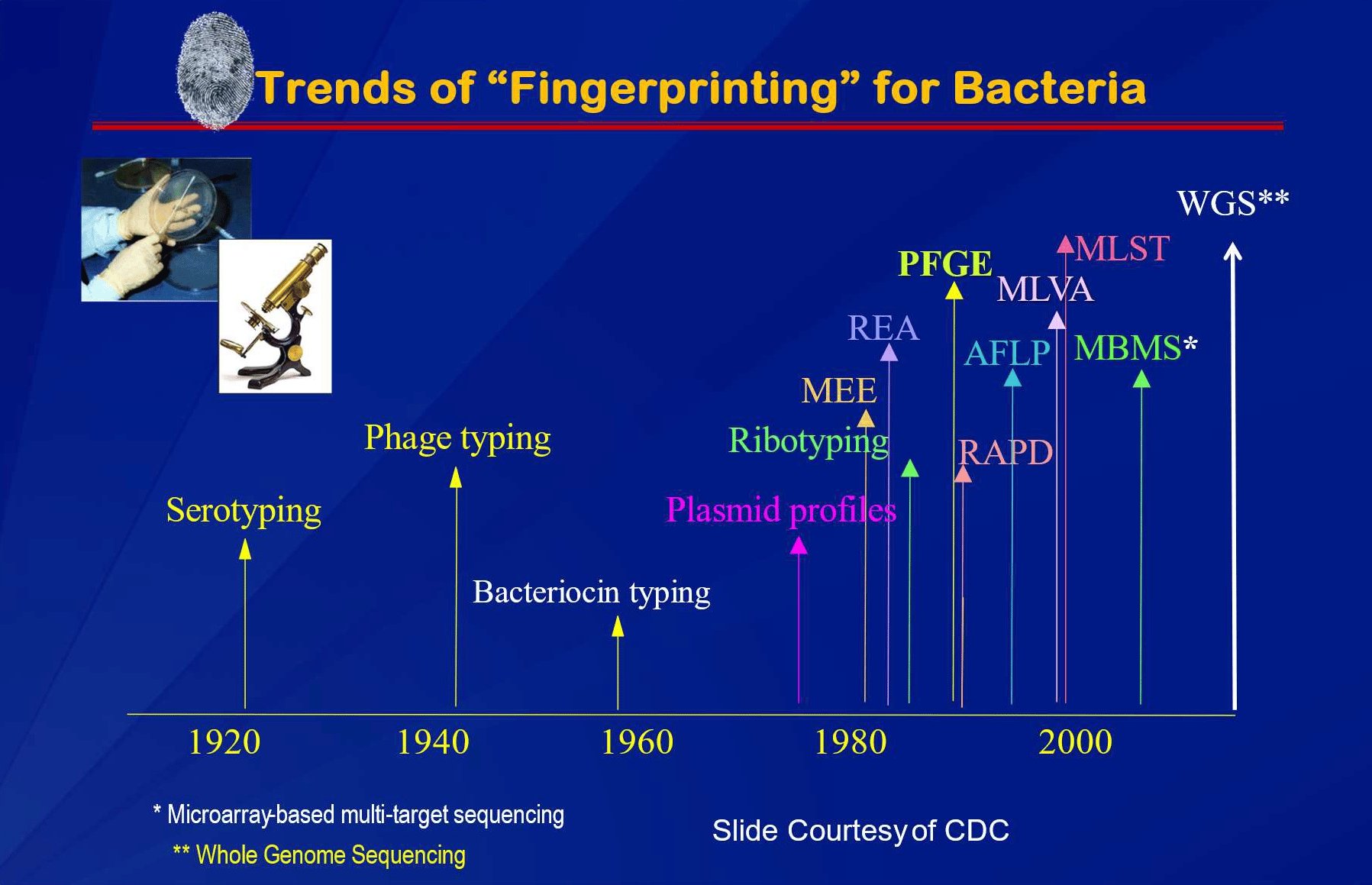
In Figure 3, the term "fingerprinting" is used. However, this term is not entirely accurate, as a fingerprint is applied to an individual human for the purpose of crime scene investigation, whereas bacteria exist as populations. When the U.S. created PulseNet, pulsed field gel electrophoresis (PFGE) was considered by CDC to be a genetic "fingerprint." This same term is now applied to the more discriminating approach, whole genome sequencing (WGS).20
However, with bacterial investigations, there are no individuals, only populations. When applied in the context of bacterial source tracking, similar "fingerprints" can, and often are, assumed to be from clonal populations. Microorganisms can mutate, however. Tenover et al.,21 discussing PFGE, suggested that certain differences from an outbreak strain may be epidemiologically related. This was based on the following assumptions:
- Isolates representing the outbreak strain are recently derived from a common ancestor
- These isolates have the same genotype
- Epidemiologically unrelated isolates will have a different genotype.
The assumptions, although practical, nevertheless begged the question, "How different is different?" Also, in some instances, epidemiologically unrelated isolates were shown to have nearly indistinguishable genotypes, due to limited genetic diversity within the species or subtype. Prior to the widespread use of WGS by regulatory agencies in the U.S., the typical interpretation of identical patterns was related to the location and intensity of the bands, as described in Tenover et al21 and adapted in Table 2.
TABLE 2. Epidemiologic Interpretation of Patterns in Outbreak Strains
With WGS, we are not dealing with band differences, but with single nucleotide polymorphism (SNP) differences, or sometimes allelic differences. Regulatory agencies have thus established "rules of thumb" for these differences, as well. For example, 20 SNP differences or less are typically used to establish the potential that isolates are epidemiologically related. Some support for this comes from a study of 5,321 L. monocytogenes isolates, which supported only a 70 percent probability of isolates with 20 or fewer SNP differences originating from the same facility.22 This probability was only 66 percent with Salmonella, also with a large number (6,351) of isolates.
Further confusing this situation is that portions of a population can be transported between widely separated geographical locations, where they may also survive, grow, and colonize. When one considers travel by airplane, an individual with a clonal population of a pathogen may, in principle, be quickly transferred to other geographies and countries, where these may result in a foodborne illness. This could happen, for example, in the context of a gastrointestinal carrier or the traveler's shoe bottoms, clothing, baggage, hair, skin, etc. By this and other means, populations of microbial clones can be transmitted far and wide.
By analogy, consider that identical human twins may live in different parts of the world and are involved in quite different activities. Perhaps one such twin is a criminal, and the other is not. What would happen if the honest twin visits the country where the dishonest twin committed a host of robberies, and the honest twin gets arrested for a minor traffic violation? Will not the honest twin suffer all sorts of undeserved but severe consequences? This consideration is far less dramatic than the case of billions or trillions of clones of an organism potentially moving throughout the earth (e.g., on passengers engaging in international air travel).
Additionally, the mutation rate of the organism may vary, depending upon the environment it is in; e.g., a microbial population growing in a high-moisture environment will result in more genetic variability than a population in a dormant state in a dry environment. Not surprisingly, there is now published evidence that some strains can be widespread geographically.23 Thus, if some L. monocytogenes related subtypes can be widespread geographically, then product contamination events could be associated with different sources, which presents a challenge to traceback investigations involving these subtypes.
Thus, the CDC has historically and wisely indicated that a plausible scenario is also necessary to establish causality, in addition to a "matching" WGS result from a factory (e.g., a drain) and a product in the marketplace. Hence, the appropriate trackback investigation is needed. In the case of WGS, the same conundrum exists as with PFGE (e.g., how different is different?). In 2019, WGS of Listeria replaced PFGE as the "fingerprinting" approach used to establish epidemiologic links between the environment and foods and clinical cases. It is often recommended that industrial manufacturers of foods perform WGS on isolates derived from their EMP. However, given the confusion and potential for misinterpretation, as described above, there appears to be no great motivation by industry for this, except in the case of post-lethality ingredient suppliers or crisis situations. For example, what CEO will countenance the chance of a recall from an L. monocytogenes positive in a drain with a WGS pattern that matches the GenomeTrakr database for a foodborne contaminant, knowing there is a 30 percent chance that these isolates are unrelated if they are within 20 SNPs?
Source tracking of selected indicators will allow for more refined approaches to control over simply using the "seek-and-destroy" philosophy that currently exists. In such cases, subtyping systems such as random amplified polymorphic DNA (RAPD),24 ribotyping, FTIR imaging of isolates, etc., could be used on selected indicators such as Listeria-like organisms (e.g., the HQA assay), or Salmonella-like organisms (e.g., hydrogen sulfide-producing thermophilic Enterobacteriaceae—HTEB assay), or Enterobacteriaceae, or coliforms, etc.9 The benefit of this enhanced approach to EMP is that one can (and should) ask questions such as, "Why and how did subtype A of an indicator organism move from one place to another in my factory?" and "What practice caused this to happen, and how can I better control that practice?" These are important questions that transcend the "seek-and-destroy" model when microbial niches recur in the plant. These techniques, short of WGS, will also allow for assumptions about transfer mechanisms from one location to another within a factory. It may be pointed out that these techniques can be used to determine if strains are different, but cannot absolutely determine if they are the same strain. Nevertheless, such approaches can be a practical tool to enhance in-plant quality systems and processes, as well as prevent further recurrence of these microbial niche areas, thereby reducing product contamination risk.
“Generally, it is much easier to track indicator organisms… than it is to track Salmonella and Listeria spp., as the incidence of such indicators should be higher than pathogens in most ready-to-eat food production facilities.”

EMP and Source Tracking Recommendations
A company should perform its own intensive environmental sampling exercise, targeting the appropriate indicator organisms in Zones 1–4 and pathogens in Zones 2–4. This is not unlike the risk assessment described in Kornacki.9 Generally, it is much easier to track indicator organisms, such as Enterobacteriaceae counts, Enterococcus spp. counts, aerobic plate counts, HTEB assays, HQA assays, etc., than it is to track Salmonella and Listeria spp., as the incidence of such indicators should be higher than pathogens in most ready-to-eat food production facilities. For example, seeking niches for the presence of high populations of quantitative indicators (e.g., Enterobacteriaceae counts), combined with the HTEB assay, could be useful for informing sample sites for future Salmonella EMPs.9 The same could be applied to enhancing Listeria EMP sites by source tracking of indicators like the HQA assay. Techniques like RAPD or repetitive sequence-based PCR (rep-PCR), applied to indicators, will also provide for a better understanding of the movement of these organisms through the plant.
A more forward-looking approach would be testing Zones 2–4 environmental samples and performing 16S sequence analysis on environmental samples, as well as shotgun metagenomic approaches to determine what pathogens (genus and species) may be present in the environment. Industry could do this through an attorney-client privilege mechanism. In this way, the presence of classical, emerging, and potential future pathogens could be determined. Then, correlations with appropriate indicator organisms can be developed for routine source tracking throughout the plant. Once appropriate indicators are developed, the microbiome approach described above need only be done infrequently. Appropriate sampling sites would be placed on a rotation throughout the plant environment, and corrective actions should be taken in response to such indicators and their subtypes, recognizing that there may be a cost savings to a well-designed and informative EMP.25
Vectoring
Vectoring is the first step in source tracking in the environment. Such a program is essential to any EMP, but should be viewed as only a first step. Should contamination reoccur at or near the initial site, then a broader investigation is needed. Source tracking will inform contamination events that transcend cleaning and sanitation, enabling the company to drill deeper into root causes and solutions.
Recommendations
Academia and government
Government regulators should recognize that requiring industry to perform WGS in the context of routine EMPs creates an unacceptable liability to industry and necessarily precludes industry from deep investigation into Zones 1–4. The government (e.g., CDC, FDA, and USDA) can do its own market basket research, using the tools of metagenomics and virulotyping to determine if other, lesser surveilled pathogens may be of concern in certain foods. Similar-coded surveys by academia, using the tools of metagenomics and virulotyping to uncover pathogens in the microbiome of food types, can also be highly useful to inform the public and industry of additional risks.
Industry
The microbial ecology of food production environments is influenced by many factors, such as air flow, drain construction,26 personnel and wheeled vehicle traffic, and design and fabrication of the facility, including intact roofs that do not leak.27 Determination of the pathogenic microbiome of a facility and development of appropriate indicator organism subtyping and pathogen assays, combined with source tracking techniques, can greatly increase the ability to move beyond simply "seek and destroy" to uncovering the "whys" and "hows" of microbial movement in the food processing facility. It will also aid in the development of programs to prohibit those practices or conditions that facilitate the movement of microorganisms throughout the food production environment.
In this way, government, academia, and industry can further reduce the incidence of foodborne contaminants in ready-to-eat foods.
References
- Scallan, E., P.M. Griffin, F.J. Angulo, R. Tauxe, and R.M. Hoekstra. "Foodborne illness acquired in the United States—Unspecified agents." Emerging Infectious Diseases 17, no. 1 (January 2011).
- Allan, J., Z. Yan, L. Genzlinger, and J.L. Kornacki. "Temperature and biological soil effects on the survival of selected foodborne pathogens on a mortar surface." Journal of Food Protection 67, no. 12 (2004): 2661–2665.
- Allan, J., Z. Yan, and J.L. Kornacki. "Surface material, temperature, and soil effects on the survival of selected foodborne pathogens in the presence of condensate." Journal of Food Protection 67, no. 12 (2004): 2666–2670.
- Reij, M.W., and E.D. Den Antrekker. "Recontamination as a source of pathogens in processed foods." International Journal of Food Microbiology 91 (2004): 1–11.
- Kornacki, J.L. "The Coming Storm in the Spice Industry." Food Safety Magazine December 2016/January 2017.
- Kornacki, J.L. "The Coming Storm in the Spice Industry, Part II: What the Industry Can Do." Food Safety Magazine February/March 2017.
- Gabis, D.A. and R.E. Faust. "Controlling microbial growth in the food processing environment." Food Technology (December 1988): 81–82, 89.
- Tompkin, R.B., L.N. Christiansen, A.B. Shaparis, R.L. Baker, and J.M. Schroeder. "Control of Listeria monocytogenes in processed meats." Food Australia 44 (1992): 370–376.
- Kornacki, J.L. "An environmental sampling approach to product risk assessment." Food Safety Magazine February/March 2014.
- Thimothe, et al. "Tracking of Listeria monocytogenes in smoked fish processing plants." Journal of Food Protection 67, no. 2 (2004): 328–341.
- Kornacki, J.L. "Chapter 7: Research during microbial food safety emergencies and contaminant investigations." In Microbiological Research and Development for the Food Industry. P.J. Taormina, Ed. CRC Press, Taylor & Francis Group, 2012.
- Kornacki, J.L. "Challenging Common STEC Assumptions." Food Safety Magazine August/September 2020.
- Kornacki, J.L. and J.B. Gurtler. "Chapter 17: Incidence and control of Listeria in food processing facilities." In Listeria, Listeriosis and Food Safety. E.T. Ryser and E.H. Marth, Eds. CRC Press, Taylor & Francis Group, 2017.
- Food Safety Preventive Controls Alliance (FSPCA). FSPCA Human Food Participant Manual V1.2: Preventive Controls for Human Food. 1st Ed. February 1, 2016. https://bookstorefspca.ifpti.org/index.php/course-materials/hazard-analysis-and-preventive-controls-for-human-foods-participant-manual.html.
- Kornacki, J.L. and D.A. Gabis. "Microorganisms and refrigeration temperatures." Dairy, Food and Environmental Sanitation (now Food Protection Trends) 10 (1990): 192–195.
- Rothschild, M. "Pasteurized Milk Linked to Yersinia Outbreak." Food Safety News. August 1, 2011. https://www.foodsafetynews.com/2011/08/pasteurized-milk-linked-to-yersina-outbreak/.
- Ackers, M.-L., S. Schoenfeld, J. Markman, M.G. Smith, M.A. Nicholson, W. DeWitt, D.N. Cameron, P.M. Griffin, and L. Slutsker. "An Outbreak of Yersinia enterocolitica O:8 Infections Associated with Pasteurized Milk." Journal of Infectious Diseases 181 (2000): 1834–1837. https://doi.org/10.1086/315436.
- Pritchard, T.J., C.M. Beliveau, K.J. Flanders, and C.W. Donnelly. "Environmental Surveillance of Dairy Processing Plants for the Presence of Yersinia Species." Journal of Food Protection 58, no. 4 (1995): 395–397.
- Gurtler, J.B, M.P. Doyle, and J.L. Kornacki, Eds. Foodborne Pathogens: Virulence Factors and Host Susceptibility. Springer, 2017.
- Carleton, H. "2019: PulseNet laboratories transition to whole genome sequencing." Centers for Disease Control and Prevention. 2019. https://archive.cdc.gov/#/details?url=https://www.cdc.gov/amd/whats-new/pulsenet-transition.html.
- Tenover, F.C., R.D. Arbeit, R.V. Goering, P.A. Mickelsen, B.E, Murray, D.H. Persing, and B. Swaminathan. "Interpreting chromosomal DNA restriction patterns produced by pulsed-field gel electrophoresis: Criteria for bacterial strain typing." Journal of Clinical Microbiology 33 (1995): 2233–2239.
- Wang, Y.U., J.B. Pettengill, A. Pightling, R. Timme, M. Allard, E. Strain, and H. Rand. "Genetic diversity of Salmonella and Listeria isolates from food facilities." Journal of Food Protection 81 (2018): 2082–2089.
- Orsi, R.H, B. Jagadeesan, L. Baert, and M. Wiedmann. "Identification of Closely Related Listeria monocytogenes isolates with no apparent evidence for a common source or location: A retrospective whole genome sequencing analysis. Journal of Food Protection 84, no. 7 (2021): 1104–1113.
- Siragusa, G., J.L. Kornacki, and N. van Loan. "Novel Approaches to Subtyping in Non-Crisis Situation." Food Safety and Microbiology Conference. Washington, D.C., December 4–6, 2022.
- Kornacki, J.L. 2021. "How and Why Environmental Monitoring Programs Add to the Bottom Line." Food Safety Magazine February/March 2021.
- Kornacki, J.L. "Airborne Contamination: A Microbiologist's Perspective." Food Safety Magazine June/July 2014.
- Kornacki, J.L. "Processing plant investigations: Practical approaches to determining sources of persistent bacterial strains in the industrial food processing environment." In The Microbiological Safety of Low Water Activity Foods and Spices. J.B. Gurtler, J.L. Kornacki, and M.P. Doyle, Eds. Springer, 2014.
Jeffrey L. Kornacki, Ph.D. is President and Senior Technical Director of Kornacki Microbiology Solutions Inc. He is also a member of the Editorial Advisory Board of Food Safety Magazine.